CARBON SKELETONS
Carbon has a unique role in the cell because of its ability to form strong covalent bonds with other carbon atoms. Thus carbon atoms can join to form:
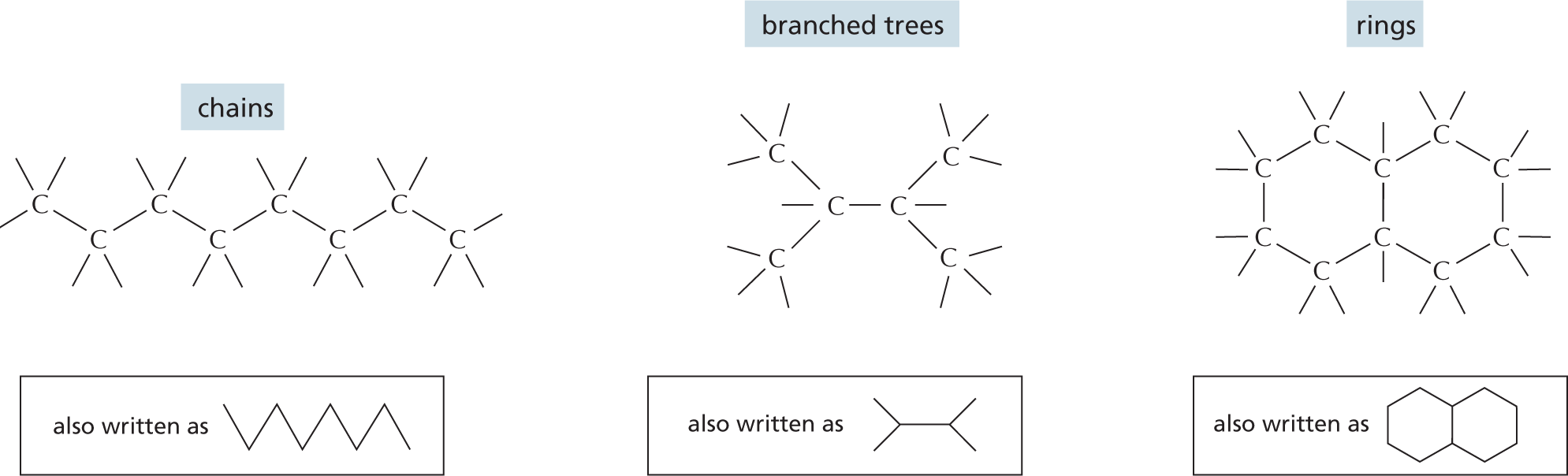
More information
Panel 1 shows carbon skeletons of chains, branched trees, and rings. An introductory text reads, carbon has a unique role in the cell because of its ability to form strong covalent bonds with other carbon atoms. Thus carbon atoms can join to form chains, branched trees, and rings as follows:
Chains are shown as an 8-carbon linear chain in which each carbon atom has two open single bonds except for the two terminal atoms, which each have three open single bonds. Chains can also be written as a triangular waveform.
Branched trees are shown as a 2-carbon linear chain in which each carbon atom is bonded to two other carbon atoms, each of which are attached to three open single bonds. The two carbon atoms in the linear chain are also attached to an open single bond. This can also be written as a horizontal line that has a V-shaped branch attached on both ends.
Rings are shown as two 6-carbon rings that are bound to each other. These two rings share two carbon atoms at the fusion site. The other carbon atoms each have two open single bonds attached to them. This can also be written as two fused hexagonal rings.
COVALENT BONDS
A covalent bond forms when two atoms come very close together and share one or more of their outer-shell electrons. Each atom forms a fixed number of covalent bonds in a defined spatial arrangement.
SINGLE BONDS: two electrons shared per bond
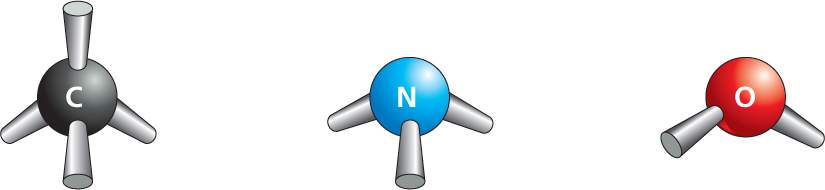
More information
Panel 2 shows covalent bond types. An introductory text reads, a covalent bond forms when two atoms come very close together and share one or more of their outer-shell electrons. Each atom forms a fixed number of covalent bonds in a defined spatial arrangement.
In single bonds, two electrons are shared per bond. Ball and stick models of carbon with 4 single bonds, nitrogen with 3 single bonds, and oxygen with two single bonds are shown.
DOUBLE BONDS: four electrons shared per bond
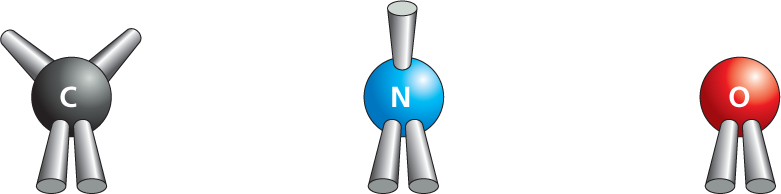
More information
In double bonds, four electrons are shared per bond. Ball and stick models of carbon with two single bonds and a double bond, nitrogen with a single bond and a double bond, and oxygen with a double bond are shown.
Accompanying text reads, the precise spatial arrangement of covalent bonds influences the three-dimensional structure and chemistry of molecules. A red colored text reads, in this review panel, we see how covalent bonds are used in a variety of biological molecules.
The precise spatial arrangement of covalent bonds influences the three-dimensional structure and chemistry of molecules. In this review panel, we see how covalent bonds are used in a variety of biological molecules.
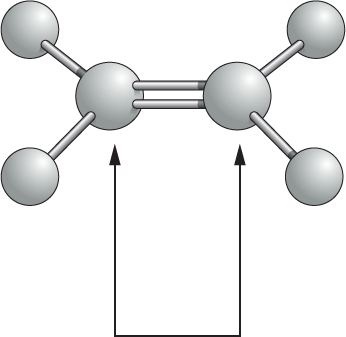
More information
Another ball and stick model shows two double bonded gray spheres, which are each single bonded to two smaller gray spheres. A double headed arrow points toward the two double bonded central spheres. Accompanying text reads, atoms joined by two or more covalent bonds cannot rotate freely around the bond axis. This restriction has a major influence on the three-dimensional shape of many macromolecules.
Atoms joined by two or more covalent bonds cannot rotate freely around the bond axis. This restriction has a major influence on the three-dimensional shape of many macromolecules.
ALTERNATING DOUBLE BONDS
A carbon chain can include double bonds. If these are on alternate carbon atoms, the bonding electrons move within the molecule, stabilizing the structure by a phenomenon called resonance.
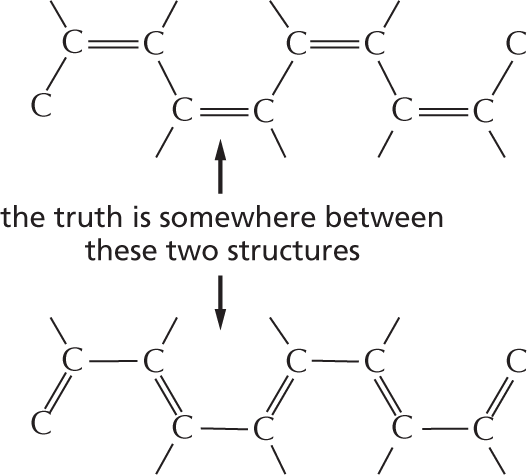
More information
Panel 3 shows alternating double bonds. An introductory text reads, a carbon chain can include double bonds. If these are on alternate carbon atoms, the bonding electrons move within the molecule, stabilizing the structure by a phenomenon called resonance.
An illustration depicts a ten carbon-chain in which C 1 and C 2, C 3 and C 4, C 5 and C 6, C 7 and C8, and C 9 and C 10 are single bonded to each other, whereas C 2 and C 3, C 4 and C 5, C 6 and C 7, and C 8 and C 9 are double bonded to each other.
Another illustration depicts a ten carbon-chain in which C 1 and C 2, C 3 and C 4, C 5 and C 6, C 7 and C8, and C 9 and C 10 are double bonded to each other, whereas C 2 and C 3, C 4 and C 5, C 6 and C 7, and C 8 and C 9 are single bonded to each other.
Alternating double bonds in a ring can generate a very stable structure.
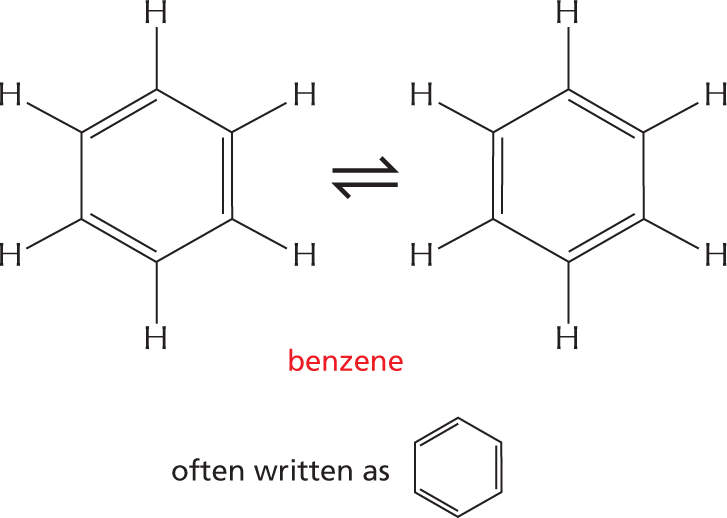
More information
A text between the two illustrations reads, the truth is somewhere between these two structures.
An equation depicts a reversible conformation between two six membered rings identified as benzene. The rings have the following configuration: C 1, C 2, C 3, C 4, C 5, and C 6 are each single bonded to a hydrogen atom; the rings have alternating double bonds. Text above the equation reads, alternating double bonds in a ring can generate a very stable structure. These rings can also be written as one hexagonal ring with alternating double bonds.
C–H COMPOUNDS
Carbon and hydrogen together make stable compounds (or groups) called hydrocarbons. Because C and H atoms have similar electronegativities, these compounds are non-polar; they therefore do not form hydrogen bonds and are generally insoluble in water.
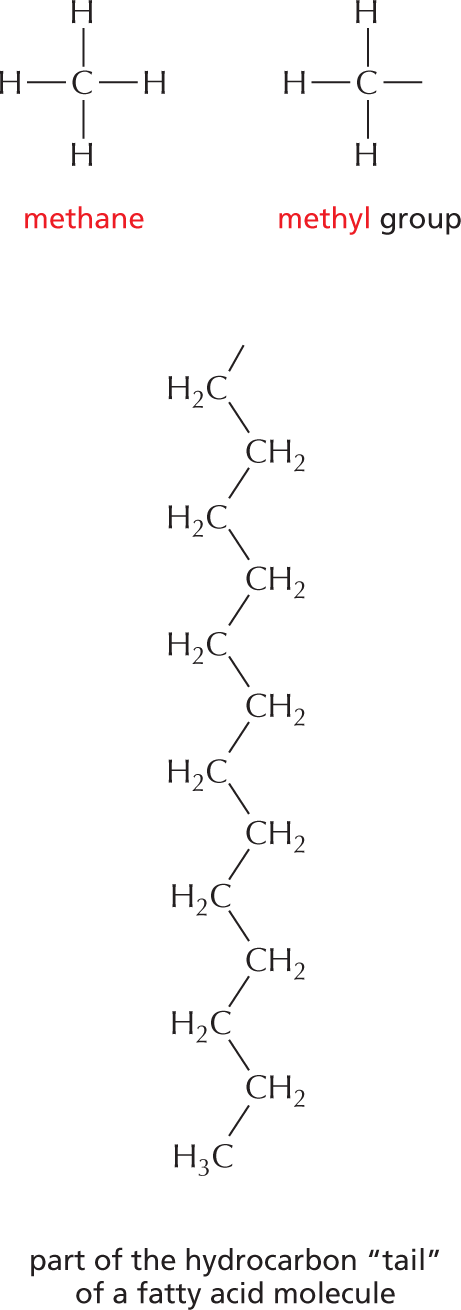
More information
Panel 4 shows hydrocarbons. An introductory text reads, Carbon and hydrogen together make stable compounds, or groups, called hydrocarbons. Because C and H atoms have similar electronegativities, these compounds are nonpolar. They therefore do not form hydrogen bonds and are generally insoluble in water.
The structural formulas of methane and a methyl group are shown. The formula of methane shows a central carbon atom single bonded to four hydrogen atoms. The formula of a methyl group shows a central carbon atom single bonded to three hydrogen atoms; it has an additional open single bond attached to it.
The structural formula of a fatty acid chain is shown alongside. The structure shows a carbon chain in which thirteen C H subscript 2 groups are single bonded to each other. A text at the bottom reads, part of the hydrocarbon double quotes tail double quotes of a fatty acid molecule.
C–O COMPOUNDS
Many biological compounds contain a carbon covalently bonded to an oxygen. For example,
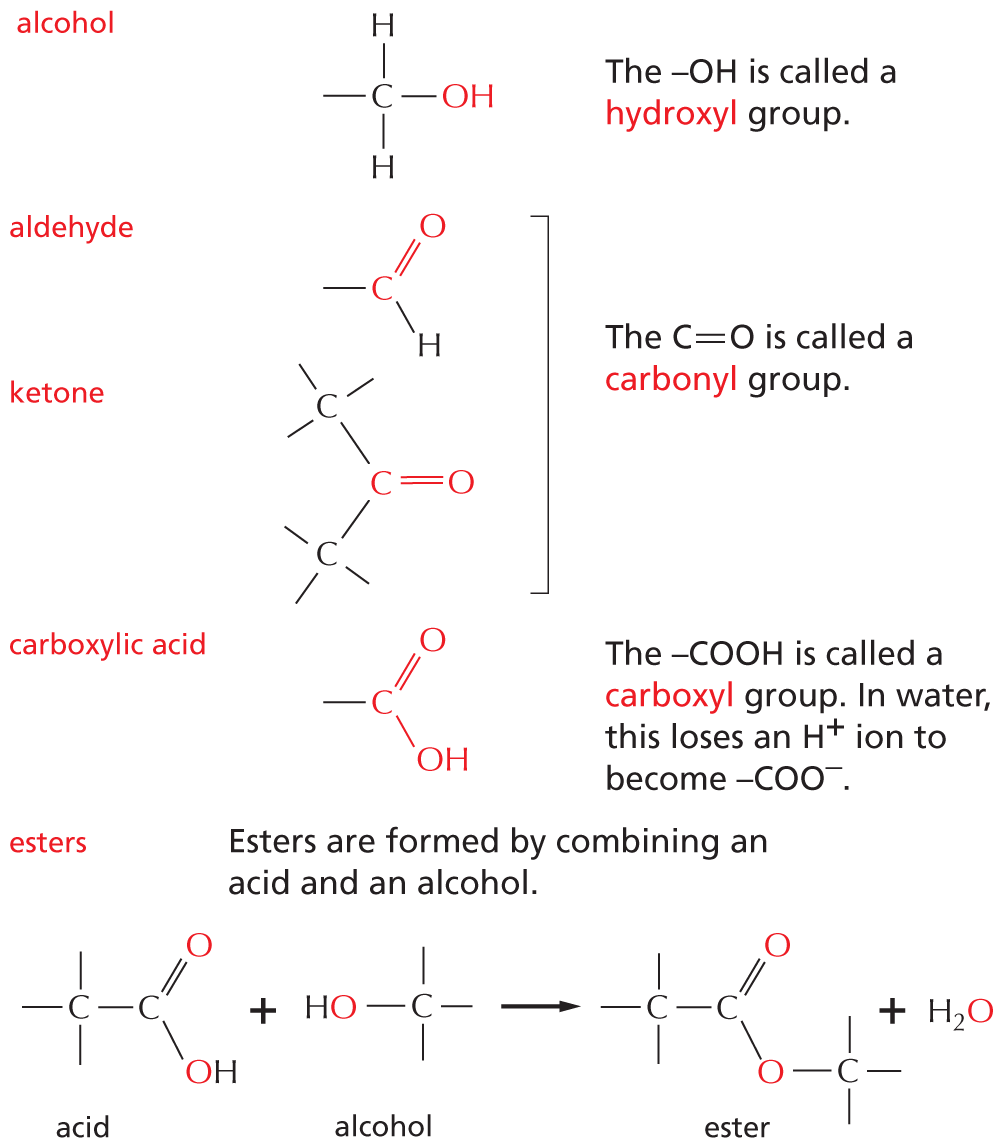
More information
Panel 5 shows compounds in which carbon is covalently bound to an oxygen. An introductory text reads, many biological compounds contain a carbon covalently bonded to an oxygen. For example,
Alcohol: A structure shows a central carbon atom single bonded to two hydrogen atoms and O H. A text beside reads, O H is called a hydroxyl group.
Aldehyde: A structure shows a central carbon atom single bonded a hydrogen atom and double bonded to an oxygen atom.
Ketone: A central carbon atom is double bonded to an oxygen and single bonded to two carbon atoms. The two carbon atoms have three more single bonds.
A text beside aldehyde and ketone reads, the C double bond O is called a carbonyl group.
Carboxylic acid: A central carbon atom is single bonded to O H and double bonded to an oxygen atom. A text beside reads, C O O H is called carboxyl group. In water, this loses an H superscript plus ion to become C O O superscript minus.
Esters: Esters are formed by combining an acid and an alcohol. A chemical reaction below shows an acid and an alcohol reacting to yield an ester and a water molecule. In acid, a central carbon atom is double bonded to an oxygen atom and single bonded to a carbon atom and O H. The carbon atom has three single bonds. In alcohol, a carbon atom is single bonded O H and has three single bonds. In ester, a central carbon atom is double bonded to an oxygen atom and single bonded to a carbon atom and an oxygen atom which is single bonded to a carbon atom. The carbon atoms have three single bonds.
C–N COMPOUNDS
Amines and amides are two important examples of compounds containing a carbon linked to a nitrogen.
Amines in water combine with an H+ ion to become positively charged.
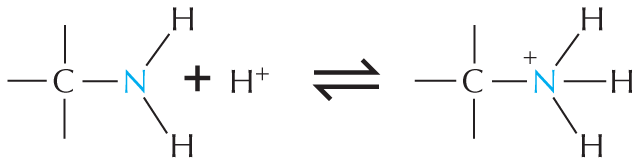
More information
Panel 6 shows amines and amides. An introductory text reads, amines and amides are two important examples of compounds containing a carbon linked to a nitrogen.
Amines in water combine with an H plus ion to become positively charged.
A structural formula shows a central nitrogen atom single bonded to two hydrogen atoms and a carbon atom reacting with a hydrogen plus ion to form a compound. This compound has a central positively charged nitrogen ion single bonded to three hydrogen atoms and a carbon atom. The reaction is shown to be reversible.
Amides are formed by combining an acid and an amine. Unlike amines, amides are uncharged in water. An example is the peptide bond that joins amino acids in a protein.
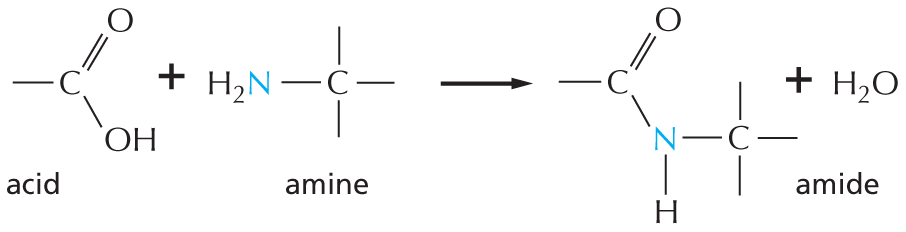
More information
Text below reads, amides are formed by combining an acid and an amine. Unlike amines, amides are uncharged in water. An example is the peptide bond that joins amino acids in a protein.
A chemical reaction is shown in which an acid reacts with an amine to form an amide and H subscript 2 O. In the acid, a carbon atom is single bonded to O H and double bonded to O. In the amine, a central carbon is single bonded to H subscript 2 N. The carbon has three single bonds. In the amide, a central nitrogen atom is single bonded to two carbon atoms and a hydrogen atom. One carbon atom is double bonded to an oxygen atom and the other carbon atom has three single bonds.
Nitrogen also occurs in several ring compounds, including important constituents of nucleic acids: purines and pyrimidines.
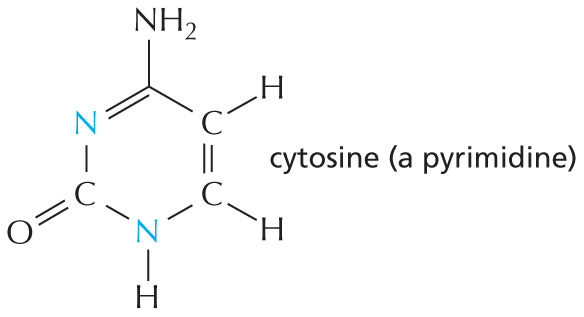
More information
Text below reads, nitrogen also occurs in several ring compounds, including important constituents of nucleic acids: purines and pyrimidines.
A six membered ring is shown in which C 1 and C 2 and C 5 and C 6 are double bonded. C 1 and C 2 are each single bonded to a hydrogen atom. C 3 has a nitrogen substituent which is single bonded to a hydrogen atom. C 4 is double bonded to an oxygen atom. C 5 is single bonded to a nitrogen atom. C 6 is single bonded to N H subscript 2. The structure is labeled cytosine, which is a pyrimidine.SULFHYDRYL GROUP
More information
Panel 7 shows sulfhydryl groups. The text reads, a carbon atom with three open single bonds and a single bonded S H group is called a sulfhydryl group. In the amino acid cysteine, the sulfhydryl group may exist in the reduced form, as a carbon atom with three open single bonds and a single bonded S H group, or more rarely in an oxidized, cross-bridging disulfide form. An example of the disulfide form is shown as a linear chain with two single bonded sulfur atoms which are each single bonded to carbon atoms; the carbon atoms each have three open single bonds.
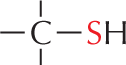
More information
Panel 7 shows sulfhydryl groups. The text reads, a carbon atom with three open single bonds and a single bonded S H group is called a sulfhydryl group. In the amino acid cysteine, the sulfhydryl group may exist in the reduced form, as a carbon atom with three open single bonds and a single bonded S H group, or more rarely in an oxidized, cross-bridging disulfide form. An example of the disulfide form is shown as a linear chain with two single bonded sulfur atoms which are each single bonded to carbon atoms; the carbon atoms each have three open single bonds.
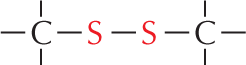
More information
Panel 7 shows sulfhydryl groups. The text reads, a carbon atom with three open single bonds and a single bonded S H group is called a sulfhydryl group. In the amino acid cysteine, the sulfhydryl group may exist in the reduced form, as a carbon atom with three open single bonds and a single bonded S H group, or more rarely in an oxidized, cross-bridging disulfide form. An example of the disulfide form is shown as a linear chain with two single bonded sulfur atoms which are each single bonded to carbon atoms; the carbon atoms each have three open single bonds.
PHOSPHATES
Inorganic phosphate is a stable ion formed from phosphoric acid, H3PO4. It is also written as P.
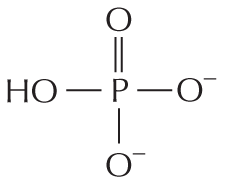
More information
Panel 8 shows phosphates. An introductory text reads, inorganic phosphate is a stable ion formed from phosphoric acid, H subscript 3 P O subscript 4. It is also written as P subscript i.
A chemical structure shows a central phosphorous atom single bonded to O H and two oxygen ions that are negatively charged and double bonded to an oxygen atom.
Phosphate esters can form between a phosphate and a free hydroxyl group. Phosphate groups are often covalently attached to proteins in this way.

More information
A text beside reads, phosphate esters can form between a phosphate and a free hydroxyl group. Phosphate groups are often covalently attached to proteins in this way.
An equation below shows a carbon single bonded to a hydroxyl group reacting with inorganic phosphate to form a phosphate ester and H subscript 2 O. In phosphate ester, a central phosphorous atom is double bonded to an oxygen atom, single bonded to two negatively charged oxygen ions, and single bonded to an oxygen that is bound to carbon. Phosphate esters can also be represented as follows: A phosphorous atom single bonded to an oxygen atom which is single bonded to a carbon atom. The carbon atom has three open single bonds attached to it.
The combination of a phosphate and a carboxyl group, or two or more phosphate groups, produces an acid anhydride. Because compounds of this type release a large amount of free energy when the bond is broken by hydrolysis in the cell, they are often said to contain a “high-energy” bond.
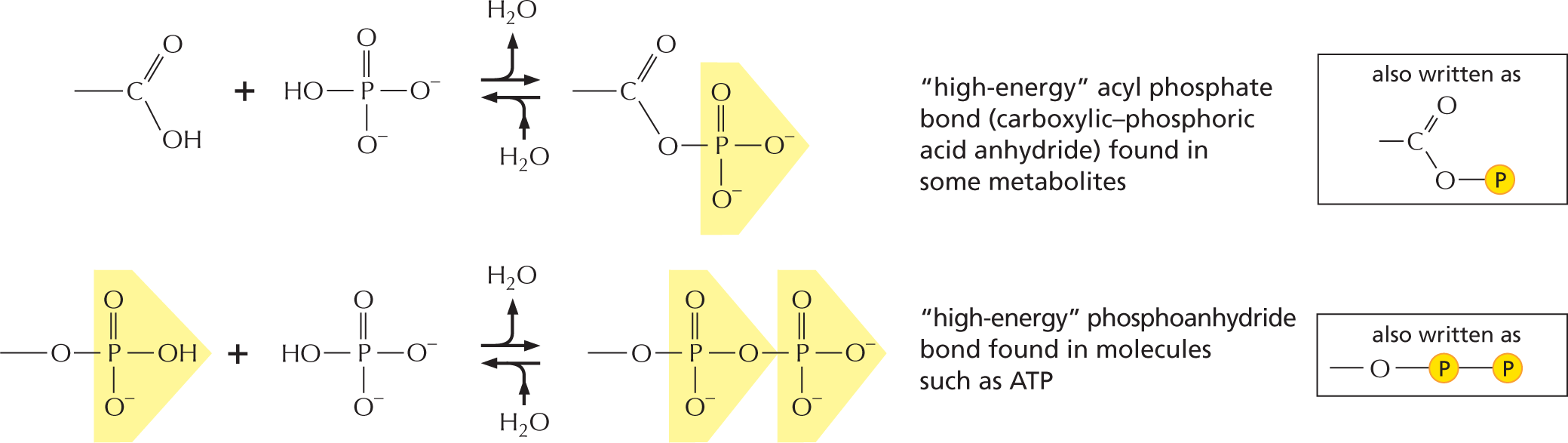
More information
The next paragraph reads, the combination of a phosphate and a carboxyl group, or two or more phosphate groups, produces an acid anhydride. Because compounds of this type release a large amount of free energy when the bond is broken by hydrolysis in the cell, they are often said to contain a high-energy bond.
An equation shows a chemical reaction, in which a carboxyl group reacts with phosphoric acid to form carboxylic-phosphoric acid anhydride. This reaction is reversible; in the forward reaction a water molecule is released and in the reverse reaction a water molecule is added. Text reads, “high-energy” acyl phosphate bond (carboxylic-phosphoric acid anhydride) found in some metabolites. The structure can also be presented as follows: a central carbon atom with a single bonded oxygen, a double bonded oxygen, and an open single bond; the single bonded oxygen is further single bonded to a phosphate group.
Another equation shows a phosphoanhydride undergoing a reversible reaction with phosphoric acid to form a compound with two phosphate groups. Accompanying text reads, “high-energy” phosphoanhydride bond found in molecules such as A T P. This can be written as: an oxygen atom single bonded to a phosphate which is further single bonded to another phosphate.