THE MAINTENANCE OF DNA SEQUENCES
The survival of an individual organism demands a high degree of genetic stability. Only rarely do the cell’s DNA-maintenance processes fail, resulting in permanent change in the DNA. Such a change is called a mutation, and it can destroy an organism if it occurs in a vital position in the DNA sequence.
Mutation Rates Are Extremely Low
The mutation rate, the rate at which changes occur in DNA sequences, can be determined directly from experiments carried out with a bacterium such as Escherichia coli—a resident of our intestinal tract and a commonly used laboratory organism (see Figure 1–38). Under laboratory conditions, an E. coli cell divides about once every 30 minutes; as a result, a single cell can generate a very large population—several billion—in less than a day. In such a population, it is possible to detect the small fraction of bacteria that have suffered a damaging mutation in a particular gene. For example, the mutation rate of a gene specifically required for cells to use the sugar lactose as an energy source can be determined by growing the cells in the presence of a different sugar, such as glucose, and testing them subsequently to see how many have lost the ability to survive on a lactose diet. The fraction of damaged genes will underestimate the actual mutation rate because many mutations are silent (for example, those that change a codon but not the amino acid it specifies or those that change an amino acid without affecting the activity of the protein coded for by the gene). After correcting for these silent mutations, one finds that bacteria display a mutation rate of about three nucleotide changes per 1010 nucleotides copied.
It is also possible to measure the germ-line mutation rate in more complex, sexually reproducing organisms such as humans. In this case, the complete genomes from a family—parents and offspring—are directly sequenced, and a careful comparison reveals that approximately 70 new single-nucleotide mutations typically arise in the germ lines of each offspring. Normalized to the size of the human genome, the mutation rate is one nucleotide change per 108 nucleotides per human generation. (This is a slight underestimate because some germ-line mutations will be lethal and will therefore be absent from progeny; however, because relatively little of the human genome carries critical information, this consideration has a negligible effect on the true mutation rate.) It is estimated that approximately 100 cell divisions occur in the germ line from the time of conception to the time of production of the eggs and sperm that go on to make the next generation. Thus, the human mutation rate, expressed in terms of cell divisions (instead of human generations), is approximately one nucleotide change per 1010 nucleotides copied.
Although E. coli and humans differ greatly in their modes of reproduction and in their generation times, when the mutation rates of each are normalized to a single round of DNA replication, they are both extremely low and within a factor of 3 of each other. We shall see later in the chapter that the basic mechanisms that ensure these low rates of mutation have been conserved since the very early history of cells on Earth.
Low Mutation Rates Are Necessary for Life as We Know It
Because many mutations are deleterious, no species can afford to allow them to accumulate at a high rate in its germ cells. Even though the observed mutation frequency is very low, it is thought to limit the number of essential genes that any organism can rely on to perhaps 30,000. More essential genes than this, and the probability that at least one critical component will suffer a damaging mutation becomes catastrophically high. By an extension of the same argument, a mutation frequency tenfold higher would limit an organism to about 3000 essential genes. In this case, evolution would have been limited to organisms considerably less complex than a fruit fly.
The cells of a sexually reproducing animal or plant are of two types: germ cells and somatic cells. The germ cells transmit genetic information from parent to offspring; the somatic cells form the body of the organism (Figure 5–1). We have seen that germ cells must be protected against high rates of mutation to maintain the species. However, the somatic cells of multicellular organisms must also be protected from genetic change to properly maintain the organized structure of the body. Nucleotide changes in somatic cells can give rise to variant cells, some of which, through “local” natural selection, proliferate rapidly at the expense of the rest of the organism. In an extreme case, the result is the uncontrolled cell proliferation that we know as cancer. This condition is due largely to an accumulation of changes in the DNA sequences of somatic cells, as discussed in Chapter 20. Any significant increase in the mutation frequency would presumably cause a disastrous increase in the incidence of cancer by accelerating the rate at which dangerous somatic-cell variants arise. Thus, both for the perpetuation of a species with a large number of genes (germ-cell stability) and for the prevention of cancer resulting from mutations in somatic cells (somatic-cell stability), multicellular organisms like ourselves absolutely depend on the remarkably high fidelity with which their DNA sequences are replicated and maintained.
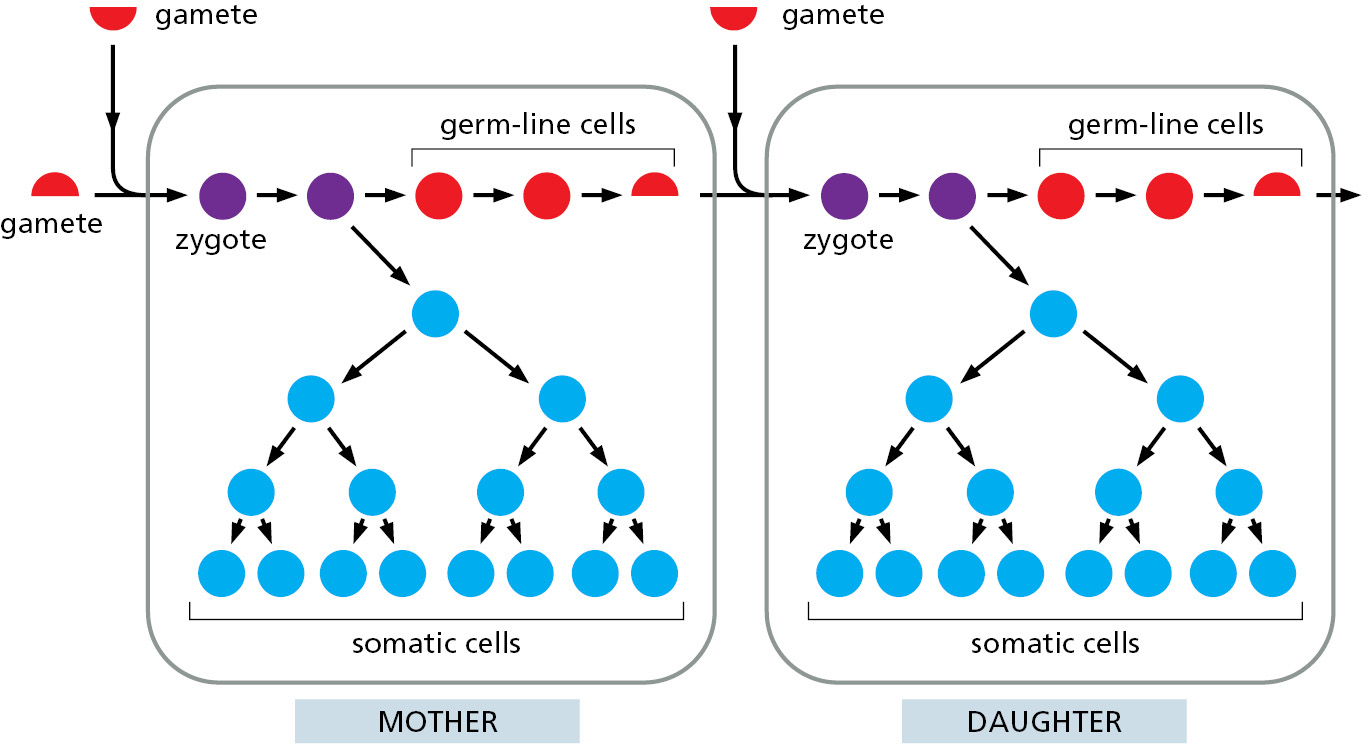
Summary
In all cells, DNA sequences are maintained and replicated with extremely high fidelity. The mutation rate, approximately one nucleotide change per 1010 nucleotides each time the DNA is replicated, is very similar for organisms as different as bacteria and humans. Because of this remarkable accuracy, the sequence of the human genome (approximately 3.1 × 109 nucleotide pairs) is unchanged or changed by only a few nucleotides each time a typical human cell divides. This allows humans to pass accurate genetic instructions from one generation to the next and also—for most of us—to avoid the changes in somatic cells that lead to cancer.
Glossary
- mutation rate
- The rate at which changes (mutations) occur in DNA sequences.
- germ cell
- A cell in the germ line of an organism, which includes the haploid gametes and their specified diploid precursor cells. Germ cells contribute to the formation of a new generation of organisms and are distinct from somatic cells, which form the body and leave no descendants.
- somatic cell
- Any cell of a plant or animal other than cells of the germ line. From Greek soma, “body.”