PEROXISOMES
Peroxisomes are major sites of oxygen utilization and are found in virtually all eukaryotic cells. They contain oxidative enzymes, such as catalase and urate oxidase, at such high concentrations that, in some cells, the peroxisomes stand out in electron micrographs because of the presence of a crystalloid protein core (Figure 12–43). The evolutionary origin of peroxisomes is not firmly established, but they are generally thought to represent a specialized offshoot of the membrane system that composes the secretory and endocytic pathways. One hypothesis is that peroxisomes are a vestige of an ancient organelle that performed all the oxygen metabolism in the primitive ancestors of eukaryotic cells. When the oxygen produced by photosynthetic bacteria first accumulated in the atmosphere, it would have been highly toxic to most cells. Peroxisomes might have lowered the intracellular concentration of oxygen, while also exploiting its chemical reactivity to perform useful oxidation reactions. According to this view, the later development of mitochondria rendered peroxisomes less critical for cellular metabolism because many of the same biochemical reactions—which had formerly been carried out in peroxisomes without producing energy—were now coupled to ATP formation by means of oxidative phosphorylation. The oxidation reactions performed by peroxisomes in present-day cells could therefore partly be those whose functions were not taken over by mitochondria.
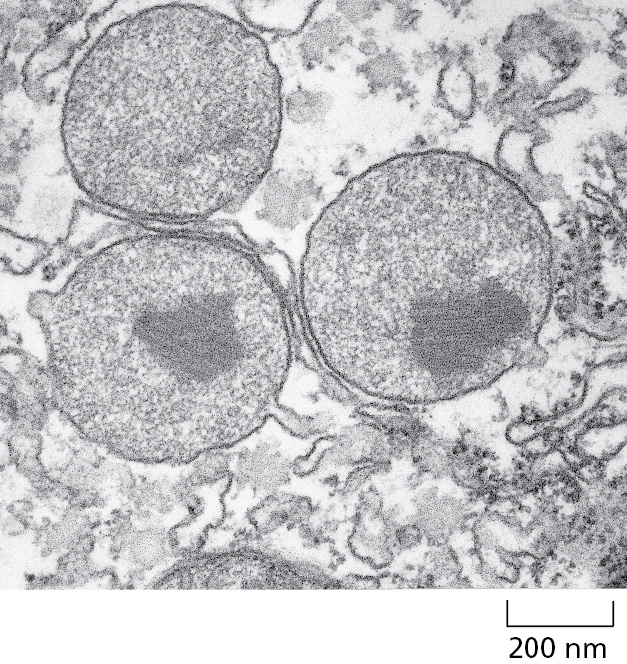
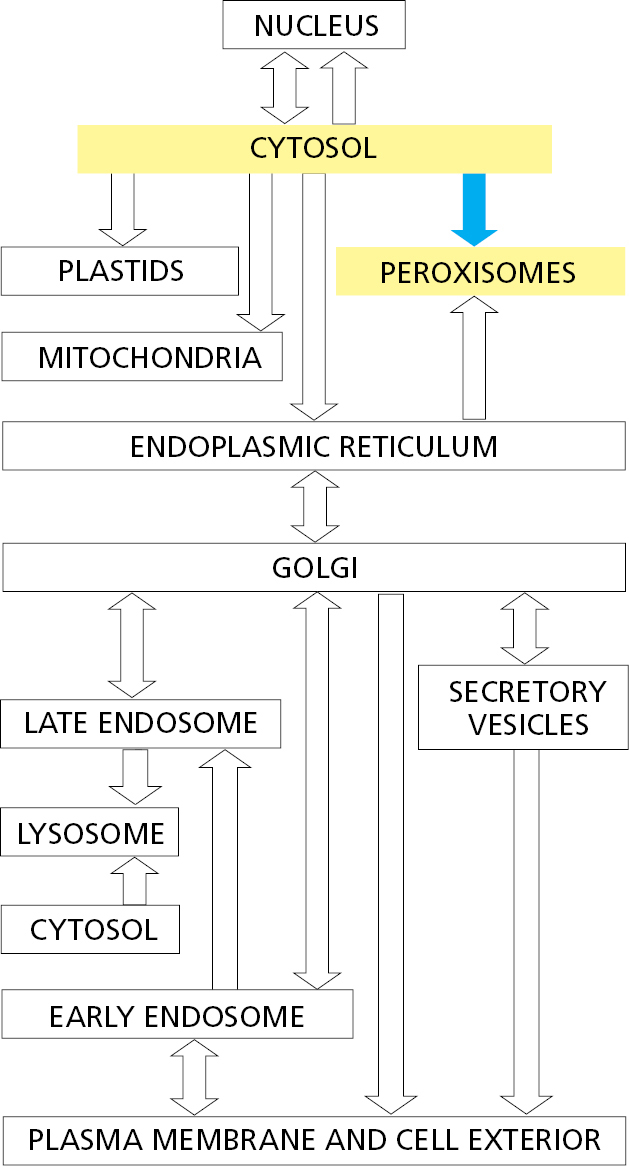
Peroxisomes Use Molecular Oxygen and Hydrogen Peroxide to Perform Oxidation Reactions
Peroxisomes are so named because they usually contain one or more enzymes that use molecular oxygen to remove hydrogen atoms from specific organic substrates (designated here as R) in an oxidation reaction that produces hydrogen peroxide (H2O2):
RH2 + O2 → R + H2O2
Catalase uses the H2O2 generated by other enzymes in the organelle to oxidize a variety of substrates—including formic acid, formaldehyde, and alcohol—by the “peroxidation” reaction: H2O2 + R′H2 → R′ + 2H2O. This type of oxidation reaction is particularly important in liver and kidney cells, where the peroxisomes detoxify various harmful molecules that enter the bloodstream. About 25% of the ethanol we drink is oxidized to acetaldehyde in this way. In addition, when excess H2O2 accumulates in the cell, catalase converts it to H2O through the reaction
2H2O2 → 2H2O + O2
A major function of the oxidation reactions performed in peroxisomes is the breakdown of fatty acid molecules. The process, called β oxidation, shortens the alkyl chains of fatty acids sequentially in blocks of two carbon atoms at a time, thereby converting the fatty acids to acetyl CoA. The peroxisomes then export the acetyl CoA to the cytosol for use in biosynthetic reactions. In mammalian cells, β oxidation occurs in both mitochondria and peroxisomes; in fungi and plant cells, however, this essential reaction occurs exclusively in peroxisomes.
An essential biosynthetic function of animal peroxisomes is to catalyze the first reactions in the formation of plasmalogens. This abundant class of phospholipids is found in all human cells but is particularly enriched in brain, where it is a major constituent of myelin (Figure 12–44). Plasmalogen deficiencies cause profound abnormalities in the myelination of nerve-cell axons, which is one reason why many peroxisomal disorders lead to neurological disease.
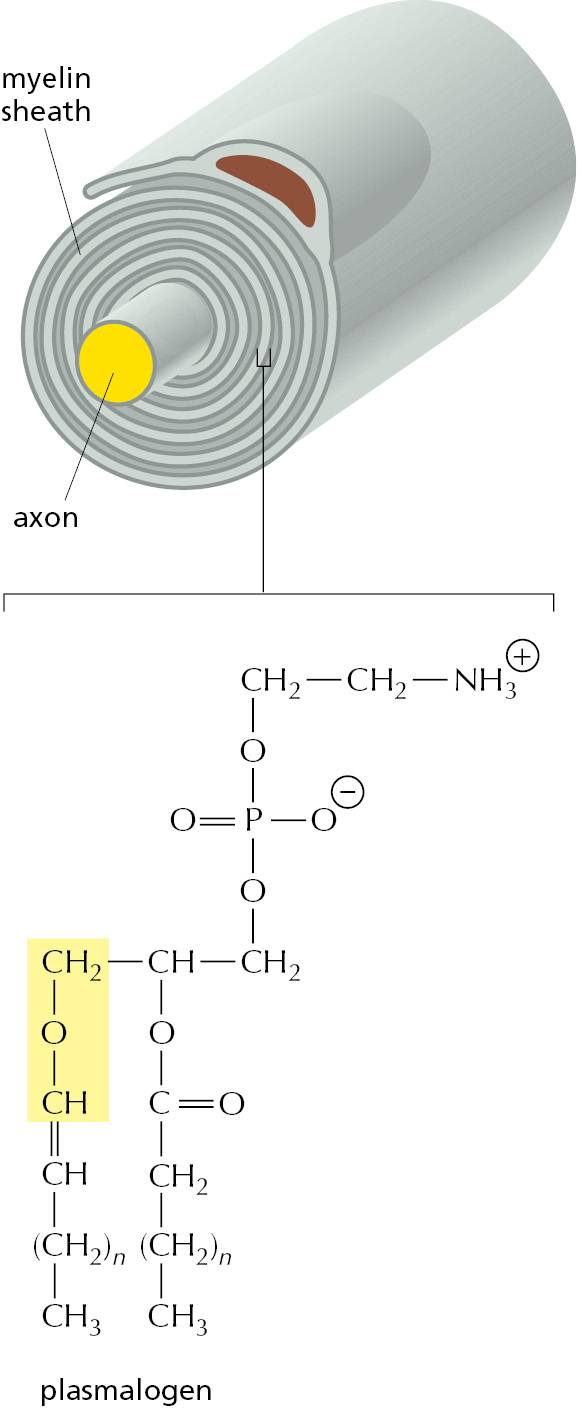
Peroxisomes are unusually diverse organelles, and even in the various cell types of a single organism they may contain different sets of enzymes. For example, most plants have two major types of peroxisomes (Figure 12–45). One is present in leaves, where it participates in photorespiration (discussed in Chapter 14). The other type of peroxisome is present in germinating seeds, where it converts the fatty acids stored in seed lipids into the sugars needed for the growth of the young plant. Because this conversion of fats to sugars is accomplished by a series of reactions known as the glyoxylate cycle, these peroxisomes are also called glyoxysomes. In the glyoxylate cycle, two molecules of acetyl CoA produced by fatty acid breakdown in the peroxisome are used to make succinic acid, which then leaves the peroxisome and is converted into glucose in the cytosol. The glyoxylate cycle does not occur in animal cells, and animals are therefore unable to convert fats into carbohydrates.
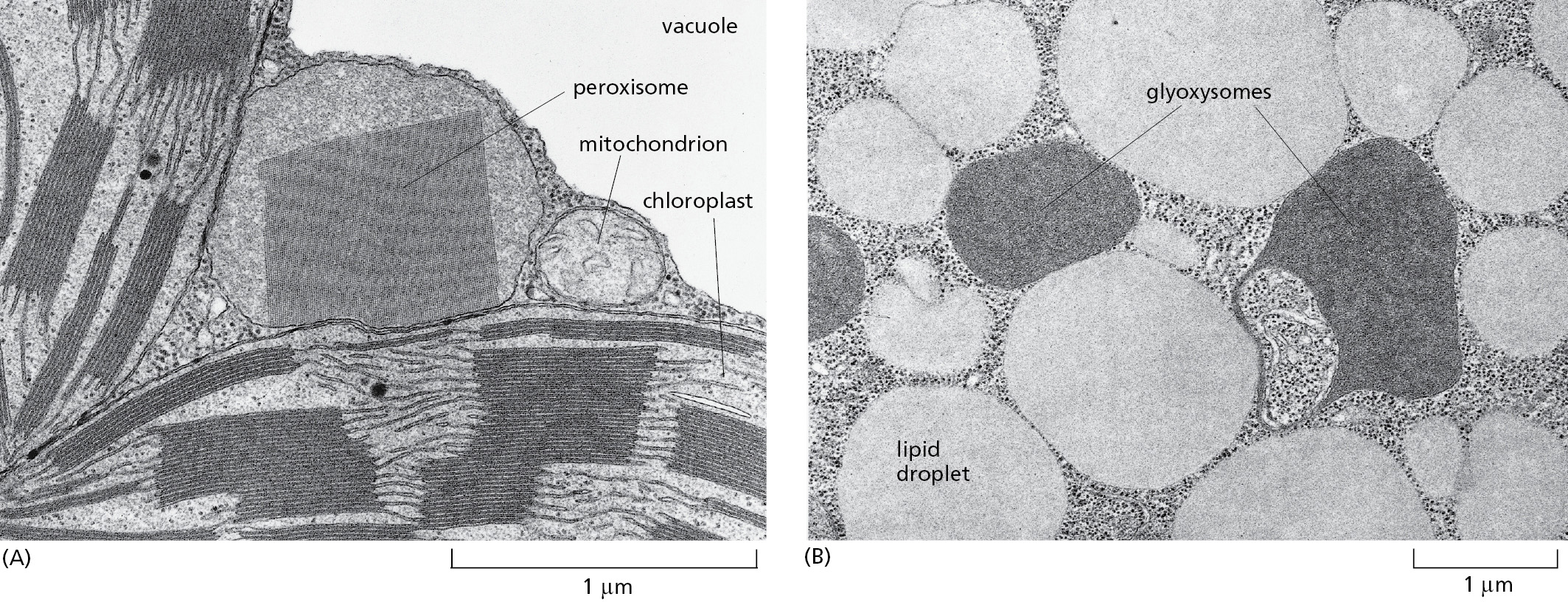
In addition to diversification across different cell types or organisms, peroxisomes can adapt to changing conditions within a cell. Yeasts grown on sugar, for example, have a few small peroxisomes. But when some yeasts are grown on methanol, numerous large peroxisomes are formed that oxidize methanol; and when grown on fatty acids, they develop numerous large peroxisomes that break down fatty acids to acetyl CoA by β oxidation.
Short Signal Sequences Direct the Import of Proteins into Peroxisomes
The proteins that compose peroxisomes are delivered by two different routes (Figure 12–46). In the first route, some of the integral membrane proteins of the peroxisomal membrane are first inserted into the ER using the ER-resident Sec61 protein translocator. These peroxisome-destined proteins are then packaged into specialized peroxisomal precursor vesicles. New precursor vesicles then fuse with one another to form a new peroxisome or fuse with an existing peroxisome to facilitate its growth. In the second route, peroxisomal proteins can be imported into preexisting peroxisomes directly from the cytosol. A specific sequence of three amino acids (Ser-Lys-Leu) located at the C-terminus of many peroxisomal proteins functions as an import signal (see Figure 12–13). Other peroxisomal proteins contain a slightly longer and partially hydrophobic signal sequence near the N-terminus. If either sequence is attached to a cytosolic protein, the protein is imported into peroxisomes.
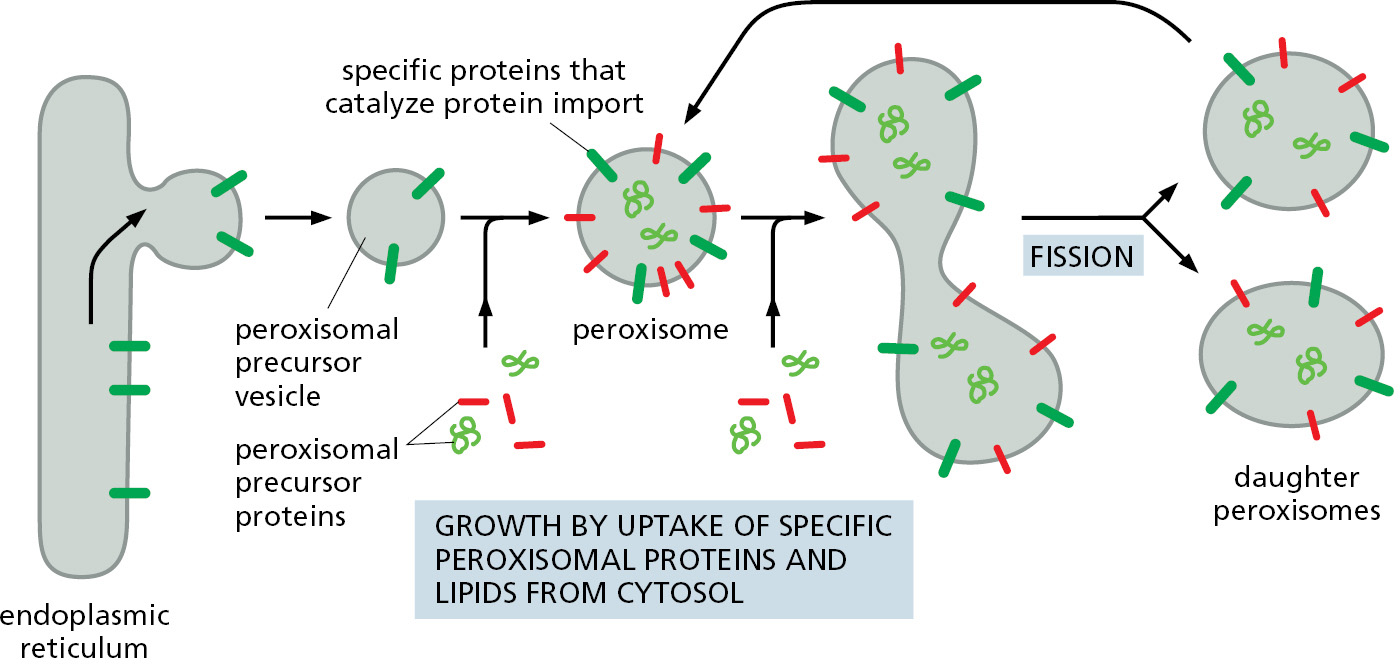
Peroxisomal protein import is driven by ATP hydrolysis and utilizes a collection of proteins, called peroxins, that catalyze the import cycle. C-terminal peroxisomal sorting signals are recognized by the peroxin Pex5 in the cytosol. This import receptor accompanies its cargo all the way into a protein translocator in the peroxisomal membrane. After cargo release inside the peroxisome, Pex5 is recycled back to the cytosol. This recycling step requires modification of Pex5 with ubiquitin, which is used as a handle by an ATPase complex composed of Pex1 and Pex6. The Pex1–Pex6 complex harnesses the energy of ATP hydrolysis to release Pex5 from peroxisomes so it can pick up the next cargo molecule. N-terminal peroxisomal signal sequences are recognized by the peroxin Pex7. The Pex7–cargo complex, together with additional accessory peroxins, appear to participate in an import cycle similar to that mediated by Pex5.
The protein translocator in the peroxisomal membrane is composed of at least six different peroxins. Unlike protein translocators in the ER, the peroxisomal translocator can transport fully folded and even oligomeric proteins across the membrane. To allow the passage of large and variably sized cargo molecules, the transporter is thought to dynamically adapt in size to the particular cargo molecules to be transported. It is not known how such a large pore can be utilized for transport without leakage of contents between the cytosol and peroxisome.
The importance of protein import into peroxisomes is demonstrated by the inherited human disease Zellweger syndrome. Mutations in any of a dozen different peroxins, the most common being Pex1, cause an impairment in peroxisomal protein import. These individuals, whose cells contain “empty” peroxisomes, accumulate very-long-chain and branched-chain fatty acids that are normally broken down in peroxisomes. Furthermore, they are deficient in plasmalogens. These metabolic impairments cause severe abnormalities in the brain, liver, and kidneys of individuals, and they die soon after birth.
Summary
Peroxisomes are specialized for carrying out oxidation reactions using molecular oxygen. They generate hydrogen peroxide, which they employ for oxidative purposes—and contain catalase to destroy the excess. All peroxisomal proteins are encoded in the cell nucleus. Some of these proteins are conveyed to peroxisomes via peroxisomal precursor vesicles that bud from the ER, but most are synthesized in the cytosol and directly imported. A specific sequence of three amino acids near the C-terminus of many of the latter proteins functions as a peroxisomal import signal that is recognized by a complementary import receptor in the cytosol. Import proceeds through a protein translocator in the peroxisomal membrane, which differs from the protein translocators in the ER in that large and fully folded proteins are imported from the cytosol without unfolding.
Glossary
- Peroxisomes
- Small membrane-bounded organelle that uses molecular oxygen to oxidize organic molecules. Contains some enzymes that produce and others that degrade hydrogen peroxide (H
- peroxins
- The proteins that form a protein translocator that participates in the import of proteins into peroxisomes.