THE TRANSPORT OF MOLECULES BETWEEN THE NUCLEUS AND THE CYTOSOL
The nuclear envelope encloses the DNA and defines the nuclear compartment. This envelope consists of two concentric membranes, which are perforated by nuclear pore complexes (Figure 12–54). Although the inner and outer nuclear membranes are continuous, they maintain distinct protein compositions. The inner nuclear membrane contains proteins that act as binding sites for the nuclear lamina, a meshwork of polymerized protein subunits called nuclear lamins. The lamin proteins are members of the intermediate filament family of cytoskeletal proteins (see Chapter 16). The lamina provides structural support for the nuclear envelope and acts as an anchoring site for chromosomes and nuclear pore complexes. The lamina is also connected to the cytoplasmic cytoskeleton via protein complexes that span the nuclear envelope, thereby providing structural links between the DNA, nuclear envelope, and cytoskeleton. The outer nuclear membrane is continuous with the membrane of the ER and is studded with ribosomes engaged in protein synthesis (see Figure 12–15). The proteins made on these ribosomes are transported into the space between the inner and outer nuclear membranes (the perinuclear space), which is continuous with the ER lumen.
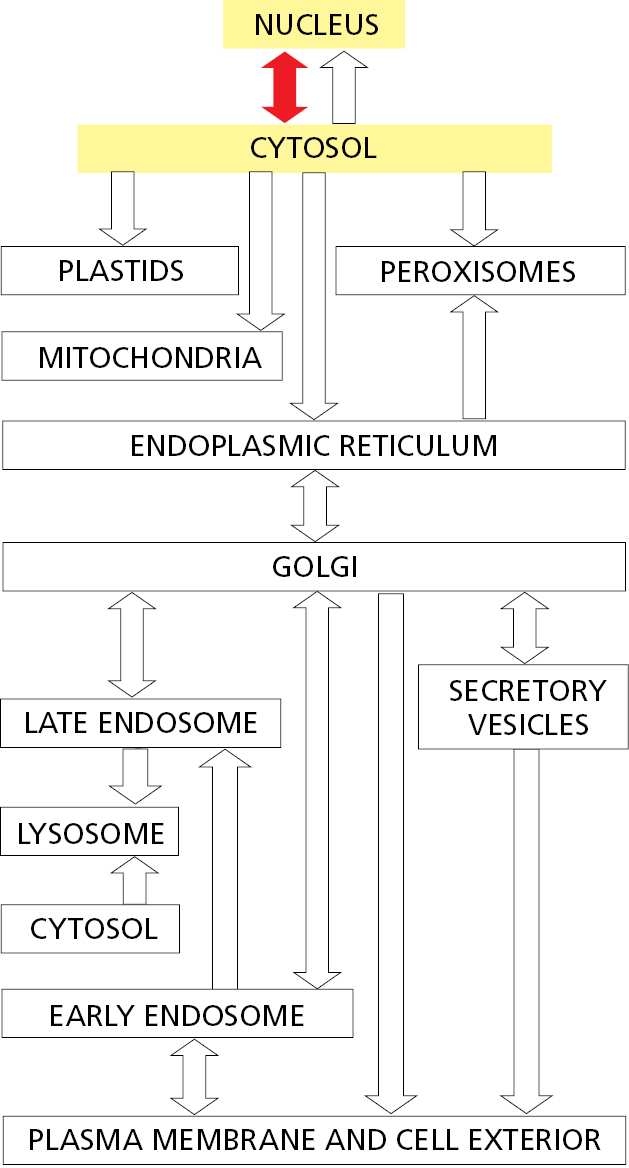
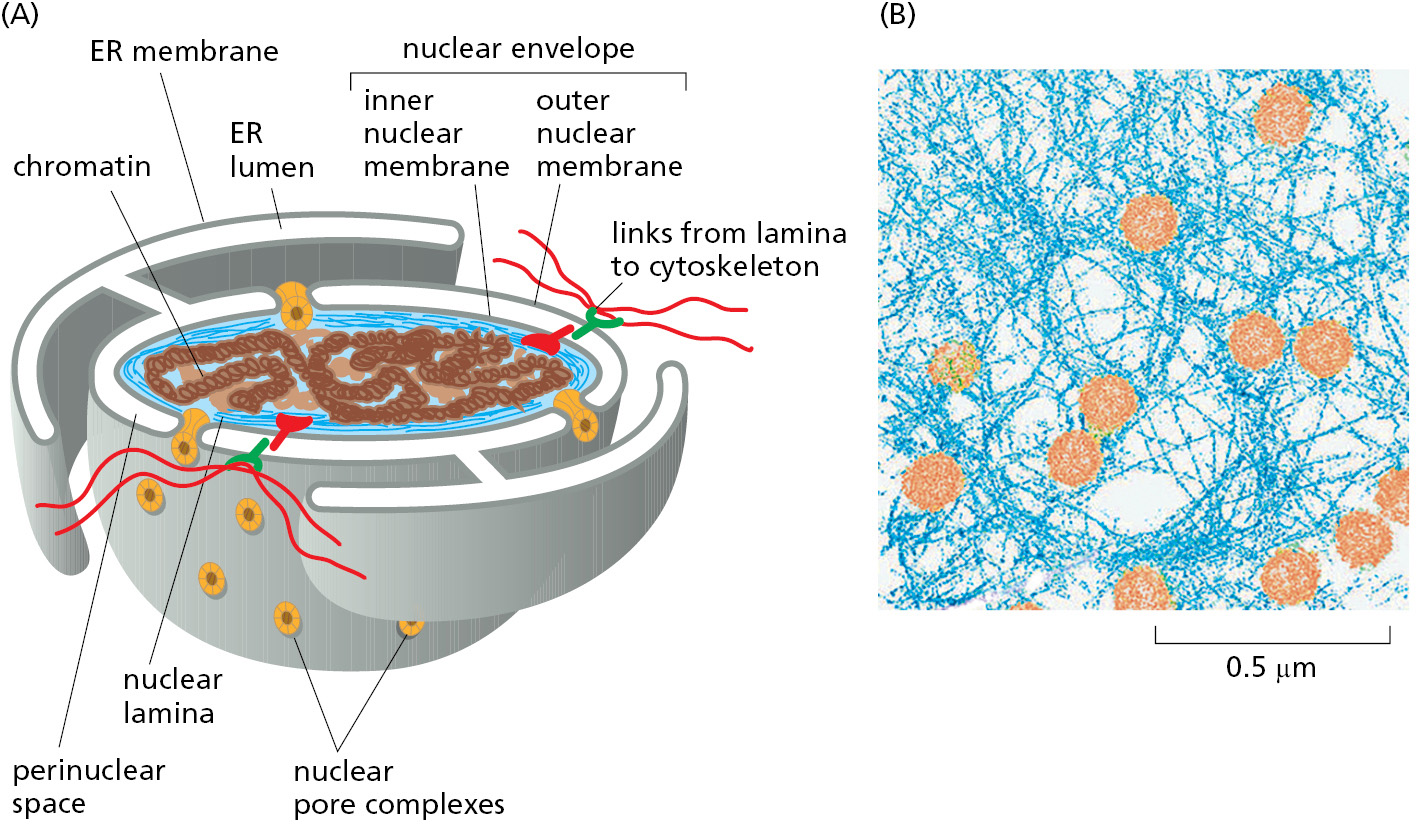
Nuclear pores conduct extensive bidirectional traffic between the cytosol and the nucleus. The many proteins that function in the nucleus—including histones, DNA polymerases, RNA polymerases, transcriptional regulators, and RNA-processing proteins—are selectively imported into the nuclear compartment from the cytosol, where they are made. At the same time, all RNAs that function in the cytosol—including mRNAs, rRNAs, tRNAs, and miRNAs—are exported after they are synthesized and processed in the nucleus. Like the import process, the export process is selective; mRNAs, for example, are exported only after they have been properly modified by RNA-processing reactions in the nucleus. In some cases, multiple selective transport steps are needed to assemble a complex structure. Ribosomes, for instance, are made from proteins that are synthesized in the cytosol, imported into the nucleus, and exported back to the cytosol only after their assembly with newly made ribosomal RNA. These pre-ribosomal particles then complete their assembly into functional ribosomes in the cytosol, with certain assembly and transport factors returning to the nucleus to help assemble the next ribosome.
Nuclear Pore Complexes Perforate the Nuclear Envelope
Large and elaborate nuclear pore complexes (NPCs) perforate the nuclear envelope in all eukaryotes. Each NPC is composed of a set of approximately 30 different proteins, or nucleoporins. NPCs display eightfold rotational symmetry, with axial symmetry of the central core. Hence, each nucleoporin is present in multiple copies, resulting in 500–1000 protein molecules in the fully assembled NPC, with an estimated mass of 66 million daltons in yeast and 125 million daltons in vertebrates (Figure 12–55). Most nucleoporins are composed of repetitive protein domains of only a few different types, which have evolved through extensive gene duplication. Some of the scaffold nucleoporins that abut the membrane (see Figure 12–55) are evolutionarily and structurally related to vesicle coat protein complexes, such as clathrin and the COPII coat (discussed in Chapter 13), which shape transport vesicles. One protein is even used as a common building block in both NPCs and vesicle coats. It appears that an ancestral membrane-bending protein that helped shape the elaborate membrane systems of eukaryotic cells evolved into a family of proteins that stabilize the sharp membrane bends at nuclear pores and budding transport vesicles.
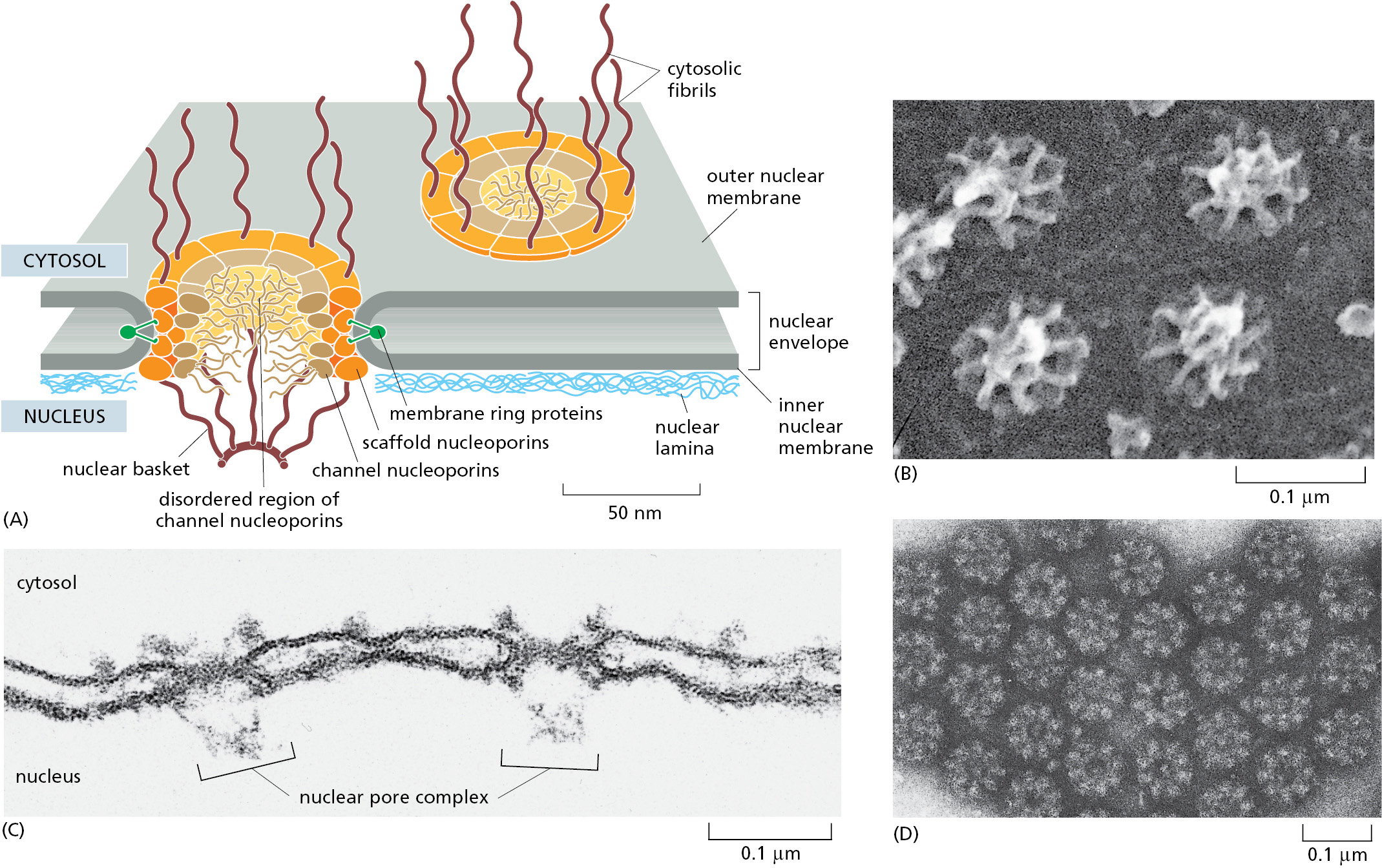
The nuclear envelope of a typical mammalian cell contains 3000–4000 NPCs, although that number varies widely, from a few hundred in glial cells to almost 20,000 in Purkinje neurons. Each NPC can transport a staggering 1000 macromolecules per second and can transport in both directions at the same time. The internal diameter of each NPC is ∼40 nm, large enough to accommodate ribosomal subunits and even viral particles. However, this enormous pore is not empty; instead, it is filled with unstructured protein regions contributed by the channel nucleoporins.
These unstructured domains contain numerous repeats of phenylalanine–glycine (FG) motifs whose weak affinity for each other creates a gel-like mesh inside the NPC. This mesh acts as a sieve that restricts the diffusion of large macromolecules while allowing smaller molecules to pass. Researchers have determined the effective size of the sieve by injecting labeled water-soluble molecules of different sizes into the cytosol and then measuring their rate of diffusion into the nucleus. Small molecules (5000 daltons or less) diffuse in so fast that we can consider the nuclear envelope freely permeable to them. The barrier is progressively restrictive to larger molecules such that proteins greater than ∼40,000 daltons or ∼5 nm in diameter cannot enter by passive diffusion.
Because many cell proteins are too large to diffuse passively through the NPCs, the nuclear compartment and the cytosol can maintain different protein compositions. Mature cytosolic ribosomes, for example, are about 30 nm in diameter and thus cannot diffuse through the NPC, confining protein synthesis to the cytosol. But how does the nucleus export newly made ribosomal subunits or import large molecules, such as DNA polymerases and RNA polymerases, which have subunit molecular masses of 100,000–200,000 daltons? As we discuss next, these and most other transported protein and RNA molecules bind to specific receptor proteins that ferry large molecules through NPCs. Even small proteins such as histones frequently use receptor-mediated mechanisms to cross the NPC, thereby increasing transport efficiency.
Nuclear Localization Signals Direct Proteins to the Nucleus
When proteins are experimentally extracted from the nucleus and reintroduced into the cytosol, even the very large ones reaccumulate efficiently in the nucleus. Sorting signals called nuclear localization signals (NLSs) are responsible for the selectivity of this active nuclear import process. The signals have been precisely defined by using recombinant DNA technology for numerous proteins that are imported into the nucleus (Figure 12–56). The most commonly used signal consists of one or two short sequences that are rich in the positively charged amino acids lysine and arginine (see Figure 12–13), with the precise sequence varying for different proteins. Some nuclear proteins contain different types of signals, some of which are not yet characterized.
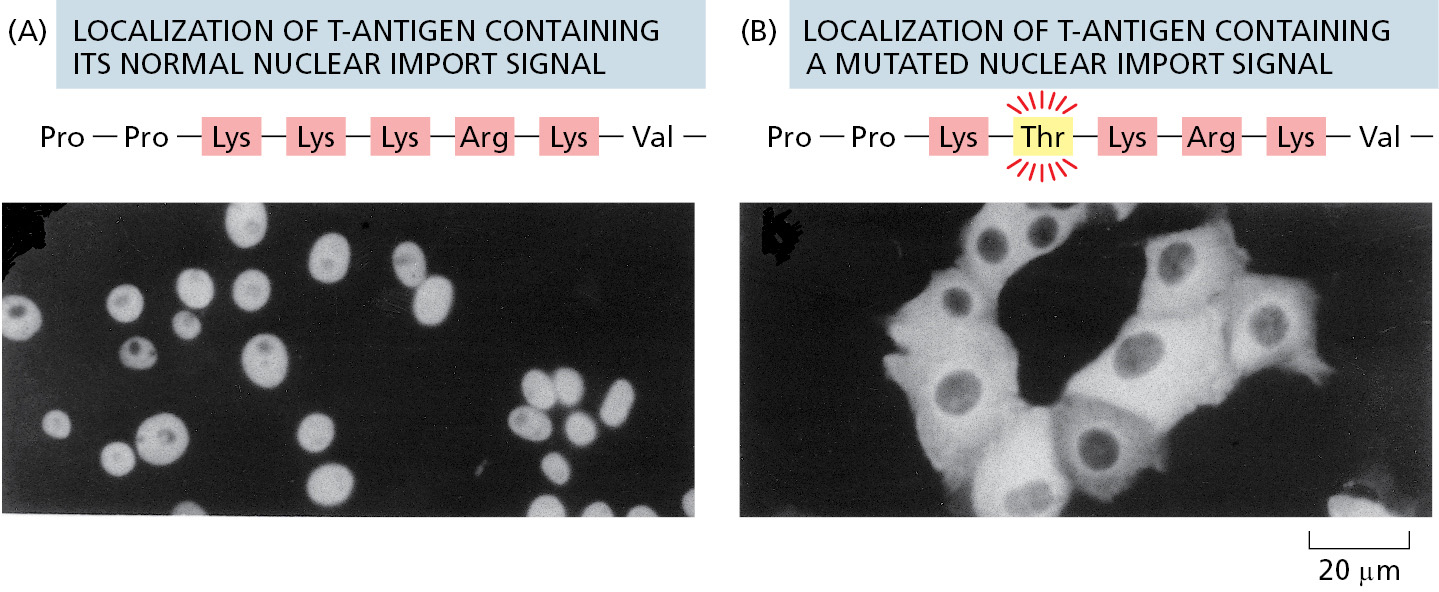
NLSs can be located almost anywhere in the amino acid sequence and are thought to form loops or patches on the protein surface. Many NLSs function even when linked as short peptides to the surface of a cytosolic protein, suggesting that the precise location of the signal within the amino acid sequence of a nuclear protein is not important. Moreover, as long as one of the protein subunits of a multicomponent complex displays a nuclear localization signal, the entire complex will be imported into the nucleus.
Macromolecular transport across NPCs differs fundamentally from the transport of proteins across the membranes of other organelles: NPC transport occurs through a large, constitutively open, mesh-filled pore, rather than through a much smaller protein translocator whose aqueous pore is typically gated by the protein being transported. For this reason, fully folded proteins and large multiprotein complexes can be transported in either direction through the nuclear pore. By contrast, transport through organellar protein translocators of the ER, mitochondria, and chloroplasts is unidirectional and usually requires the protein to be extensively unfolded.
One can visualize the transport of nuclear proteins through NPCs by coating tiny colloidal gold particles with a nuclear localization signal, injecting the particles into the cytosol, and then following their fate by electron microscopy (Figure 12–57). The particles first arrive at the tentacle-like fibrils that extend from the scaffold nucleoporins at the rim of the NPC into the cytosol, and then proceed through the center of the NPC. This observation illustrates that NLSs impart the ability of large particles to navigate through the otherwise impermeable diffusion barrier posed by the disordered mesh inside the nuclear pore.
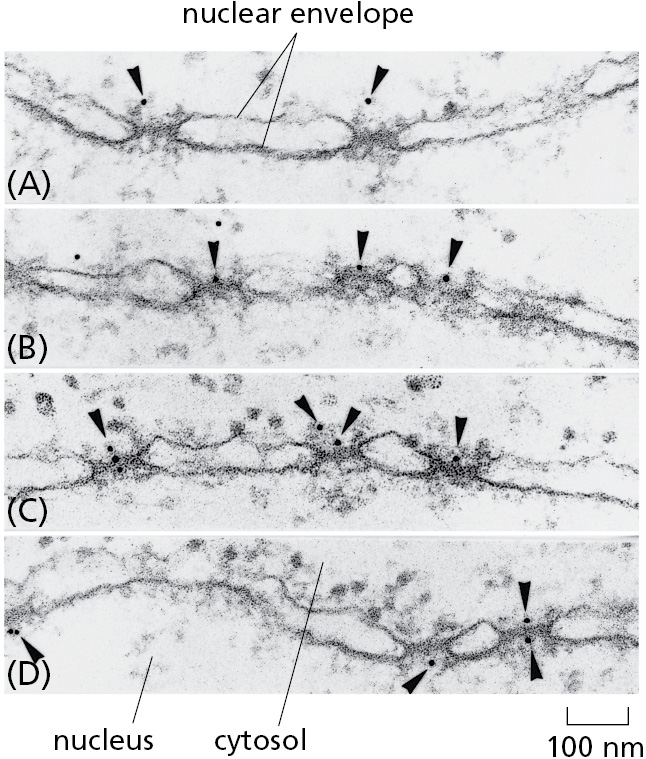
Nuclear Import Receptors Bind to Both Nuclear Localization Signals and NPC Proteins
To initiate nuclear import, nuclear localization signals must be recognized by nuclear transport receptors. Most of these receptors are part of a large family of proteins called karyopherins. In yeast, there are 14 genes encoding karyopherins; in animal cells, the number is significantly larger. Karyopherin family members that mediate nuclear import are called nuclear import receptors, while those for nuclear export (discussed later) are called nuclear export receptors. Each import receptor can bind and transport the subset of cargo proteins containing the appropriate nuclear localization signal (Figure 12–58A). Nuclear import receptors sometimes use adaptor proteins that form a bridge between the import receptors and the nuclear localization signals on the proteins to be transported (Figure 12–58B). Some adaptor proteins are structurally related to nuclear import receptors, suggesting a common evolutionary origin. By using a variety of import receptors and adaptors, cells are able to recognize the broad repertoire of nuclear localization signals that are displayed on nuclear proteins.
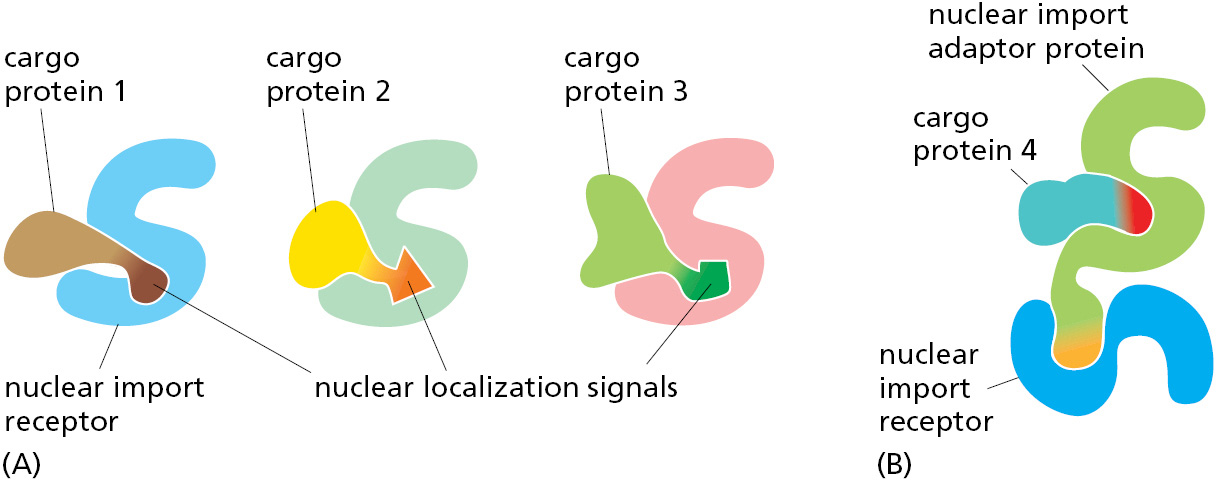
The import receptors are soluble cytosolic proteins that contain multiple low-affinity binding sites for the FG repeats found in the unstructured domains of several nucleoporins. The FG repeats in the fibrils of cytosol-facing nucleoporins serve to initially recruit import receptors and their bound cargo proteins to NPCs. The import receptors can then bind the FG repeats that form the mesh inside the nuclear pore to disrupt interactions between the repeats. In this way, the receptor–cargo complex locally dissolves the gel-like mesh and can diffuse into and within the NPC pore (Figure 12–59).
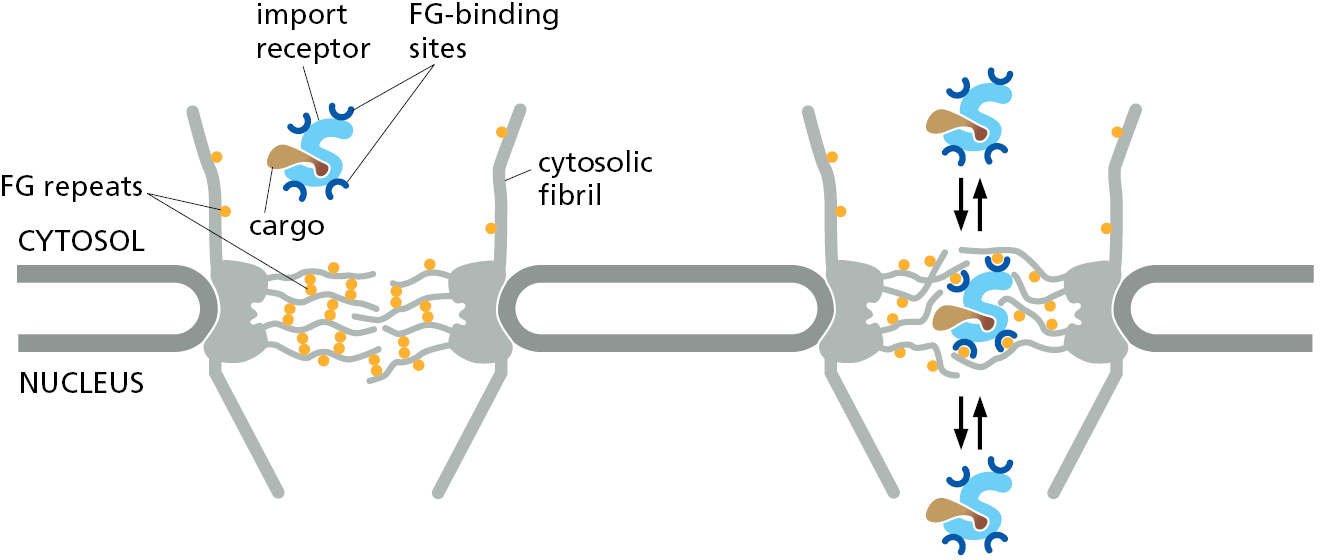
It is possible to re-create in a test tube a gel consisting of unstructured polypeptides containing FG repeats. This gel displays restricted diffusion of inert cargoes in a size-dependent manner similar to diffusion through NPCs. Diffusion into this artificial gel is more than 1000-fold faster for cargoes bound to an import receptor. At this rate, a cargo in complex with an import receptor could traverse the distance across an NPC in a few milliseconds, consistent with the rate of transport observed in cells. It is important to realize that in this model, diffusion is not directional; instead, the import receptor simply accelerates diffusion to provide cargo access to the nuclear compartment. As we will see, it is the selective dissociation of cargo only on the nuclear side of the NPC that confers directionality to the import process. The import receptor then returns back to the cytosol for transport of the next cargo.
The Ran GTPase Imposes Directionality on Nuclear Import Through NPCs
The import of nuclear proteins through NPCs concentrates specific proteins in the nucleus and thereby increases order in the cell. The cell fuels this ordering process by harnessing the energy of GTP hydrolysis by the GTPase Ran, which is required for both nuclear import and export.
Like other GTPases, Ran is a molecular switch that can exist in two conformational states, depending on whether GDP or GTP is bound (Figure 3–63). Two Ran-specific regulatory proteins trigger the conversion between the two states: a cytosolic GTPase-activating protein (GAP) triggers GTP hydrolysis and thus converts Ran-GTP to Ran-GDP, and a nuclear guanine nucleotide exchange factor (GEF) promotes the exchange of GDP for GTP and thus converts Ran-GDP to Ran-GTP. Because Ran GAP is located in the cytosol and Ran GEF is located in the nucleus, the cytosol contains mainly Ran-GDP, and the nucleus contains mainly Ran-GTP (Figure 12–60A). The partitioning of the GAP and GEF between the cytosol and nucleus in a cell is due to their preferential association with the cytosolic cytoskeleton and nuclear chromatin, respectively.
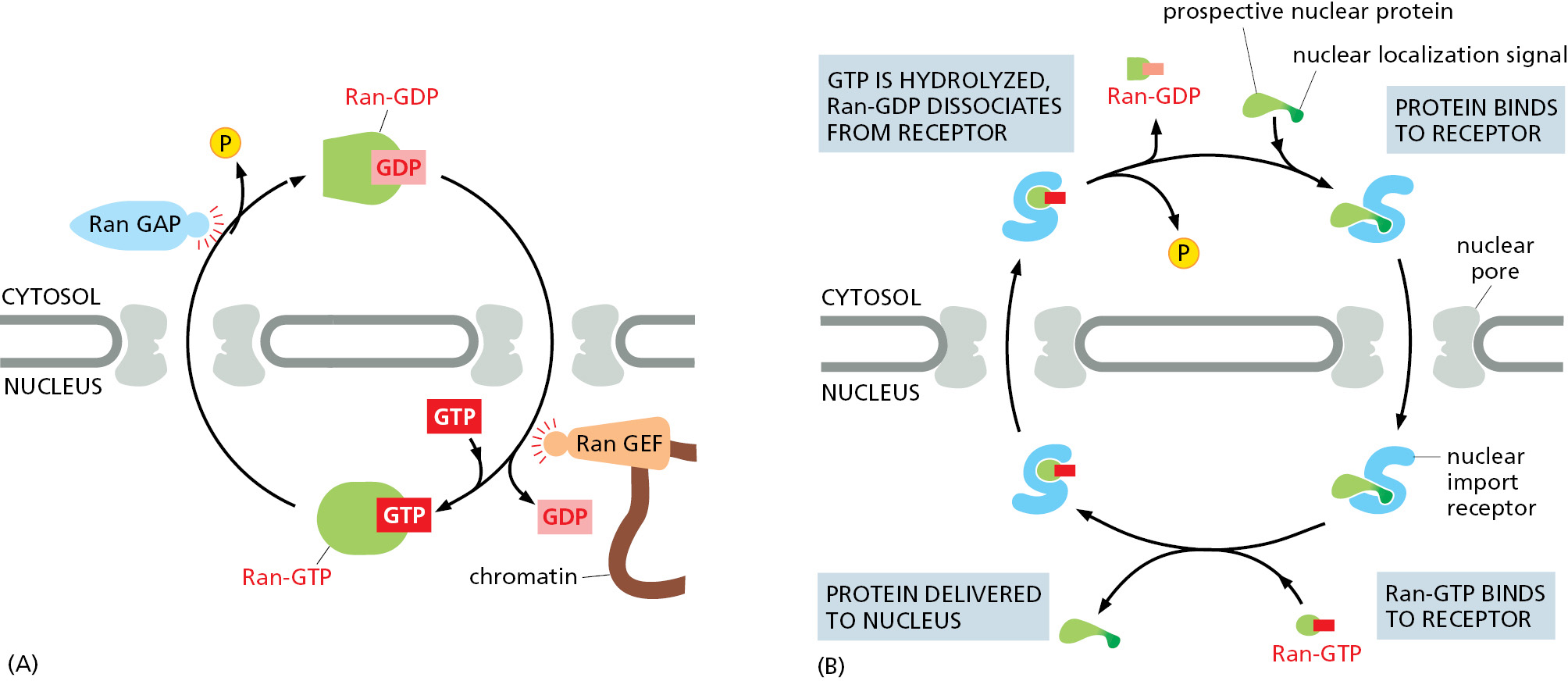
The gradient of the two conformational forms of Ran drives nuclear transport in the appropriate direction. Import receptors, facilitated by FG-repeat binding, accelerate diffusion through the mesh inside the NPC channel. When an import receptor reaches the nuclear side of the pore complex, Ran-GTP binds to it and causes the receptor to release its cargo (Figure 12–60B). Because this occurs only on the nuclear side of the pore where the Ran-GTP concentration is high, the import process becomes rectified (that is, unidirectional), even though diffusion of the cargo–import receptor complex through the pore is governed by random back-and-forth diffusion.
Having discharged its cargo in the nucleus, the empty import receptor with Ran-GTP bound is transported back through the pore complex by the same mechanism of facilitated diffusion. When the complex of Ran-GTP and the import receptor reaches the cytosol, Ran GAP triggers Ran-GTP to hydrolyze its bound GTP. The resulting Ran-GDP lacks affinity for the import receptor, releasing it for another cycle of nuclear import. Thus, Ran-GDP permits cargo binding in the cytosol, while Ran-GTP stimulates cargo discharge in the nucleus, thereby imparting directionality to the import process.
Nuclear Export Works Like Nuclear Import, but in Reverse
The nuclear export of large molecules, such as new ribosomal subunits and RNA molecules, occurs through NPCs and also depends on a selective transport system. The transport system relies on nuclear export signals on the macromolecules to be exported. Export receptors bind to both the export signal, either directly or via an adaptor, and to NPC proteins to guide their cargo to the cytosol. As might be expected from the structural and evolutionary similarity of import receptors and export receptors, the import and export transport systems work in similar ways but in opposite directions: the import receptors bind their cargo molecules in the cytosol, release them in the nucleus, and are then exported to the cytosol for reuse, while the export receptors function in the opposite fashion (Figure 12–61).
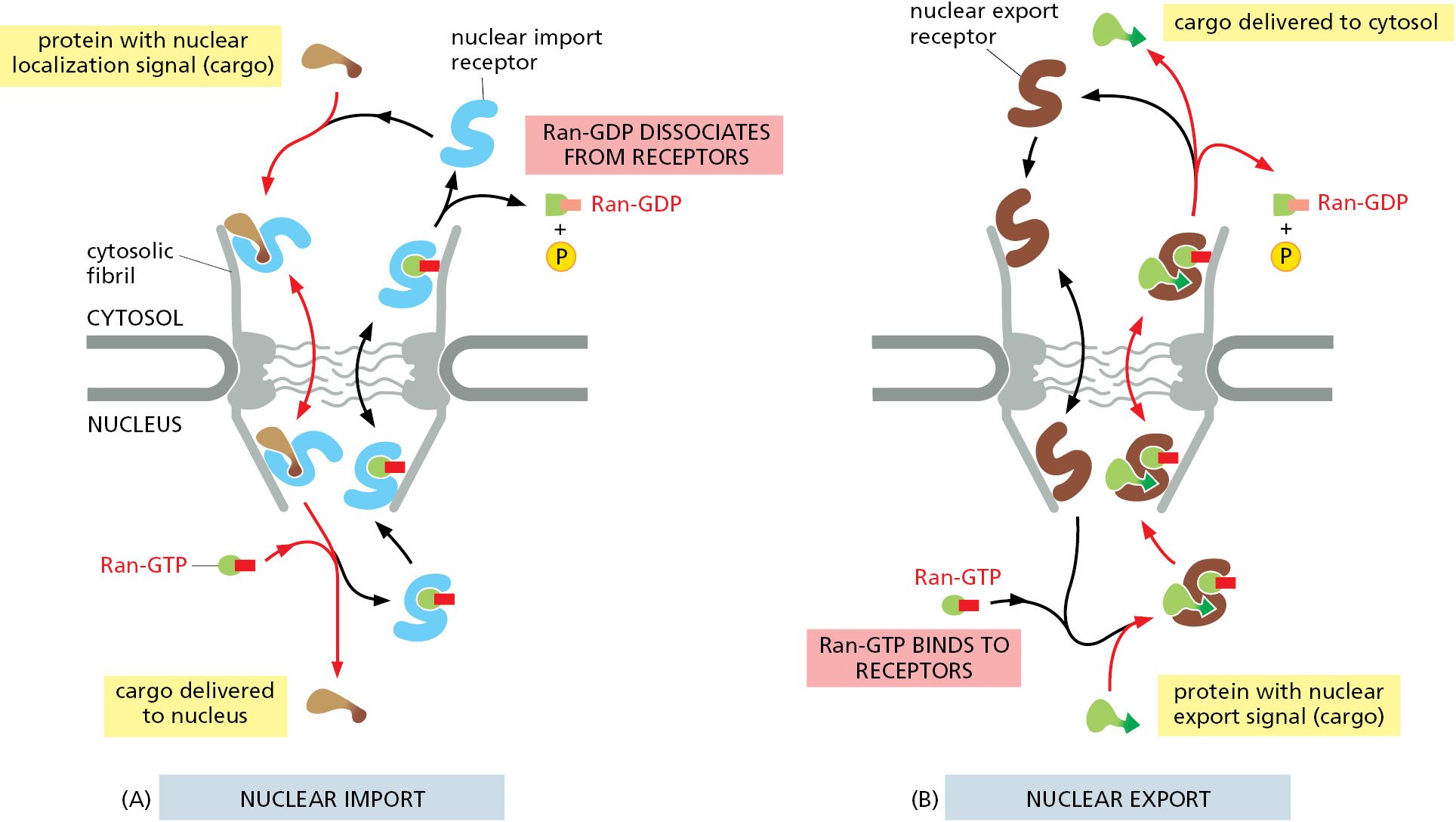
The ability of export receptors to work in reverse derives from the way they interact with the Ran GTPase. Ran-GTP in the nucleus promotes cargo binding to the export receptor, rather than promoting cargo dissociation as in the case of import receptors. Once the export receptor moves through the pore to the cytosol, it encounters Ran GAP, which induces the receptor to hydrolyze its GTP to GDP. As a result, the export receptor flips its conformation and releases both its cargo and Ran-GDP in the cytosol. Free export receptors and free Ran-GDP use the nuclear import pathway to enter the nucleus and complete the cycle.
As we discuss in detail in Chapter 6, cells control the export of RNAs from the nucleus. snRNAs, miRNAs, and tRNAs bind to nuclear export receptors, and they use the Ran-GTP gradient to fuel the transport process. By contrast, the export of mRNAs out of the nucleus uses a different mechanism that does not use export receptors or the Ran GTPase system. Instead, the spliced and processed mRNA is assembled with several nuclear RNA-binding proteins, some of which can bind the nuclear side of NPCs and others that bind FG repeats (see Figure 6–40). This export-competent mRNA ribonucleoprotein (mRNP) complex can then navigate through the FG repeat mesh within the NPC. A helicase complex that resides on the cytosolic side of NPCs uses the energy of ATP hydrolysis to strip several proteins from the mRNP, including the FG repeat–binding protein. This prevents the exported mRNA from reentering the NPC, making the export process unidirectional. The stripped RNA-binding proteins are rapidly imported back to the nucleus (using the import receptor and Ran GTPase system) for another round of transport.
Transport Through NPCs Can Be Regulated by Controlling Access to the Transport Machinery
Some proteins continually shuttle back and forth between the nucleus and the cytosol. This can happen if a protein is small enough to diffuse through the nuclear pore but contains an import or export signal that constantly retrieves it to the nucleus or cytosol. Other proteins contain both nuclear localization signals and nuclear export signals. The relative rates of their import and export determine the steady-state localization of such shuttling proteins: if the rate of import exceeds the rate of export, a protein will be located mainly in the nucleus; conversely, if the rate of export exceeds the rate of import, a protein will be located mainly in the cytosol. Thus, changing the rate of import, export, or both, can change the location of a protein.
As discussed in Chapter 7, cells control the activity of some transcription regulators by keeping them out of the nucleus until they are needed there (Figure 12–62); similarly, cells can control the translation of certain mRNAs by retaining them in the nucleus until their protein products are needed. In many cases, cells control transport by regulating nuclear localization and export signals—turning them on or off, often by phosphorylation of amino acids close to the signal sequences (Figure 12–63). Other transcription regulators are bound to inhibitory cytosolic proteins that either anchor them in the cytosol (through interactions with the cytoskeleton or specific organelles) or mask their nuclear localization signals so that they cannot interact with nuclear import receptors. An appropriate stimulus releases the transcription regulatory protein from its cytosolic anchor or mask, and it is then transported into the nucleus.
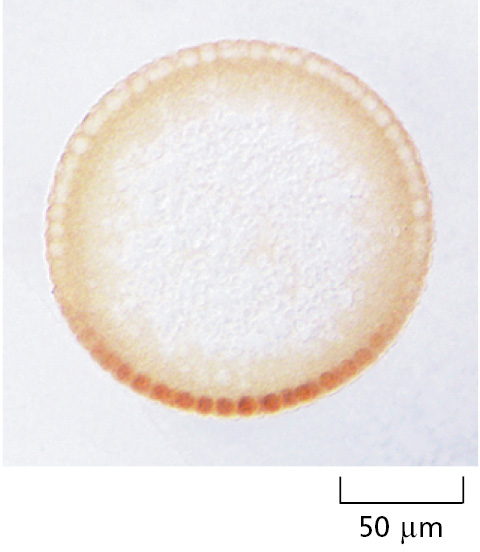
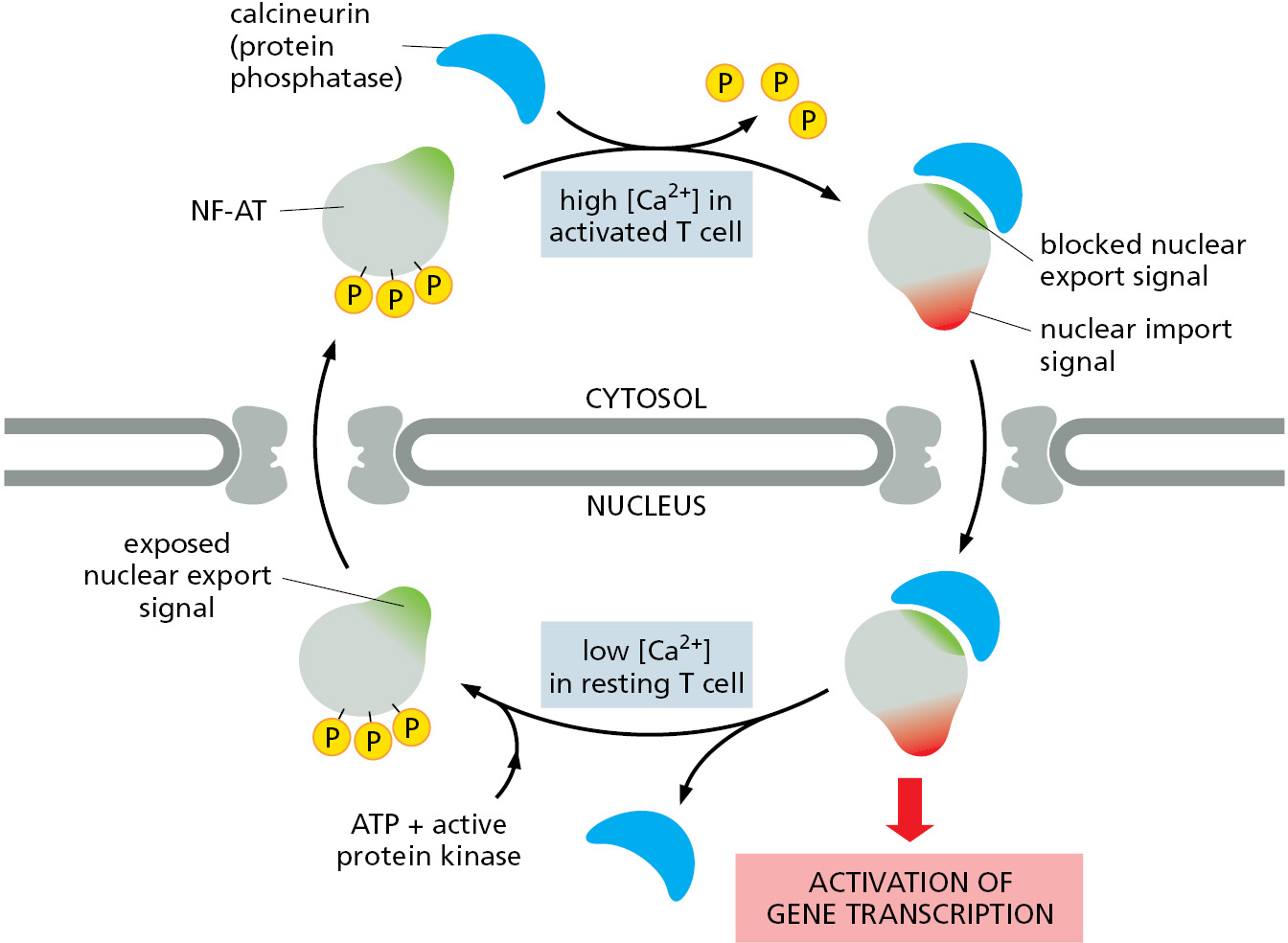
One important example is the latent transcription regulatory protein that controls the transcription of genes involved in cholesterol metabolism. The protein is made and stored in an inactive form as a transmembrane protein in the ER. When a cell is deprived of cholesterol, the protein is transported from the ER to the Golgi apparatus where it encounters specific proteases that cleave off the cytosolic domain, releasing it into the cytosol. This domain is then imported into the nucleus, where it activates the transcription of genes required for both cholesterol uptake and synthesis (Figure 12–64). Earlier in this chapter, we discussed a similar mechanism that controls the activation of the ATF6 arm of the unfolded protein response (see Figure 12–36).
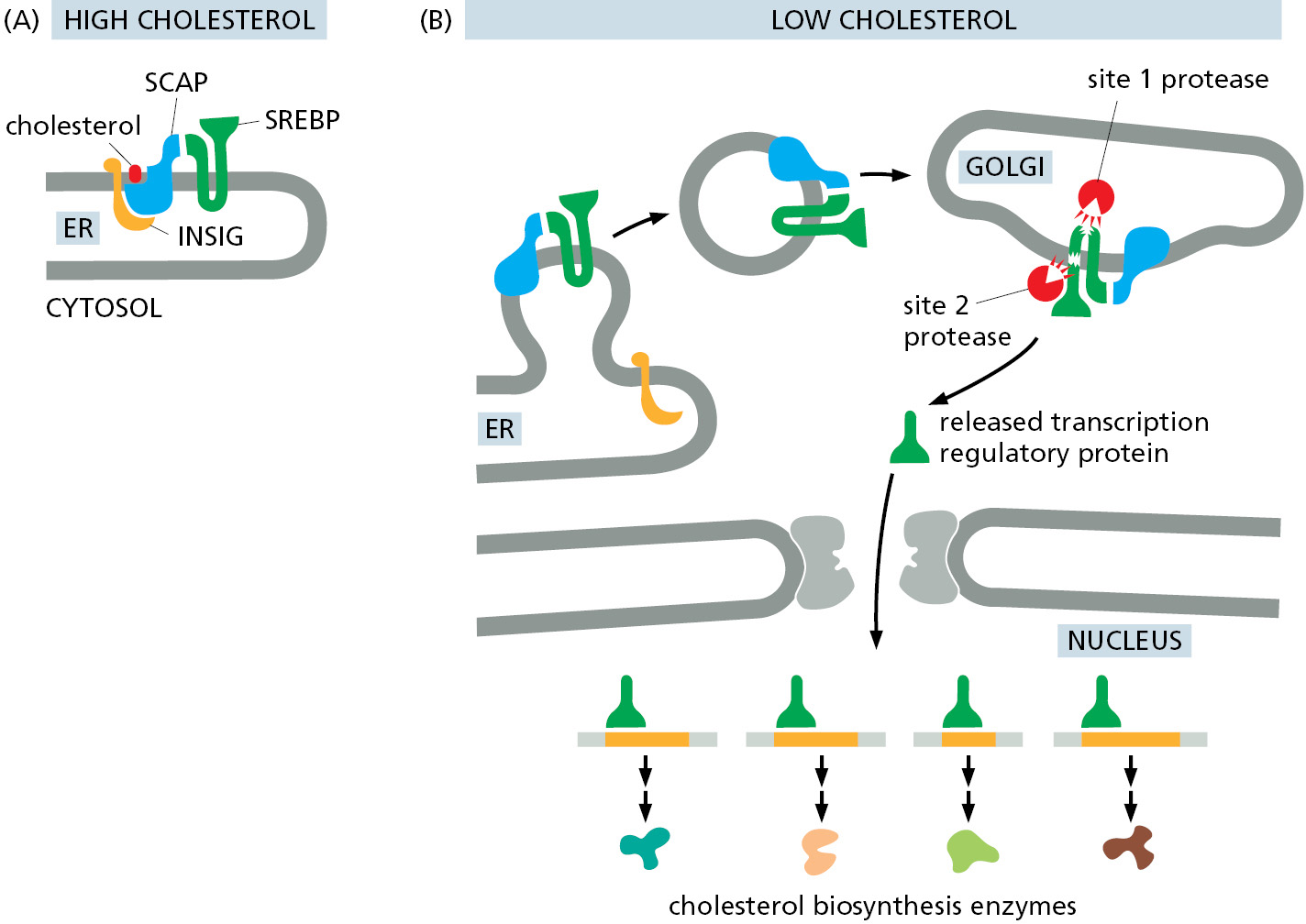
The Nuclear Envelope Disassembles and Reassembles During Mitosis
In animal cells, the nuclear envelope is dismantled during mitosis so that microtubules can access the replicated chromosomes for segregation between the two daughter cells (discussed in Chapter 17). At the end of mitosis, the nuclear envelope reassembles, and the asymmetrical distribution of cellular contents between the cytosol and nucleus is reestablished. The major structures that must be reversibly disassembled are the nuclear lamina, the NPCs, and the membranes of the nuclear envelope.
The dismantling process is initiated by the cyclin-dependent kinase (Cdk) that is activated at the onset of mitosis (discussed in Chapter 17). Cdk phosphorylates nucleoporins, lamins, and inner nuclear membrane proteins to disrupt their interactions with each other and with chromatin. During this process, some NPC proteins become bound to nuclear import receptors, which play an important part in the reassembly of NPCs at the end of mitosis. Nuclear envelope membrane proteins—no longer tethered to the pore complexes, lamina, or chromatin—disperse throughout the ER membrane. The dynein motor protein, which moves along microtubules (discussed in Chapter 16), is recruited to the outer nuclear membrane early in mitosis and exerts a pulling force. Transmembrane proteins that tether the outer nuclear membrane to the inner nuclear membrane and lamina help transduce this force and pull the nuclear envelope off the chromatin. Together, these processes break down the barriers that normally separate the nucleus and cytosol, and the nuclear proteins that are not bound to membranes or chromosomes intermix completely with the proteins of the cytosol (Figure 12–65).
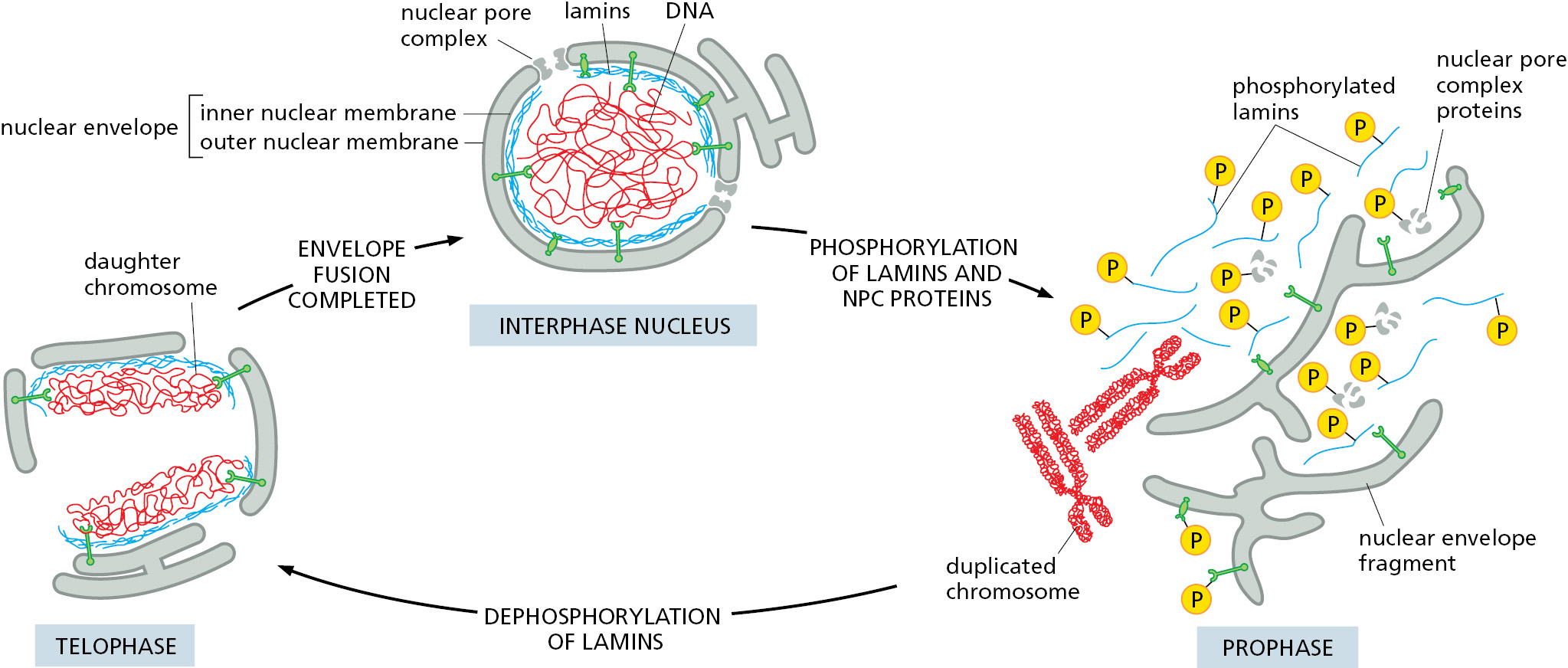
One protein that remains bound to chromatin even after the nuclear envelope breaks down is Ran GEF. This means Ran molecules close to chromatin are mainly in their GTP-bound conformation. By contrast, Ran molecules further away are in their GDP-bound conformation because of the action of cytosolic Ran GAP. As a result, the chromosomes in mitotic cells are surrounded by a cloud of Ran-GTP, which is important for assembling the mitotic spindle that segregates chromosome into the newly forming daughter cells (discussed in Chapter 17). After chromosome segregation, Cdk is inactivated, allowing dephosphorylation of nucleoporins, lamins, and nuclear membrane proteins. This triggers reassembly of the nuclear envelope on the surface of the complete set of chromosomes in each daughter cell. The positional marker for recruitment of nuclear envelope components to chromosomes is the surrounding cloud of Ran-GTP.
Ran-GTP releases the NPC proteins from nuclear import receptors in proximity to the chromosomes. The free NPC proteins attach to the chromosome surface, where they assemble into new NPCs. At the same time, dephosphorylated lamins bind again to chromatin and recruit ER membranes via the inner nuclear membrane proteins that reside within them. The ER progressively wraps around the entire group of chromosomes until the ER forms a sealed nuclear envelope, engulfing the chromosomes and proteins bound to them (Movie 12.7). The newly formed inner nuclear envelope is closely applied to the surface of the chromosomes, is enriched for inner nuclear membrane proteins, and excludes all proteins except those initially bound to the mitotic chromosomes, thus conferring a high level of selectivity to the engulfment process. Because Ran-GTP is inside the nucleus and Ran-GDP remains outside, unidirectional import of proteins that contain nuclear localization signals can occur through NPCs. In this way, the nuclear protein content is replenished, while all other large proteins, including ribosomes, are kept out of the newly assembled nucleus.
Summary
The nuclear envelope consists of an inner and an outer nuclear membrane that are connected with each other at perforations formed by nuclear pore complexes (NPCs). The outer nuclear membrane is continuous with the ER membrane, and the space between the inner and outer nuclear membranes is continuous with the ER lumen. RNA molecules, which are made in the nucleus, and ribosomal subunits, which are assembled there, are exported to the cytosol; in contrast, all the proteins that function in the nucleus are synthesized in the cytosol and are then imported. The extensive traffic of materials between the nucleus and cytosol occurs through NPCs, which provide a direct passageway across the nuclear envelope. The interior of NPCs contains a mesh of unstructured proteins that allows passage of small molecules but imposes a diffusion barrier that requires large macromolecules to be actively transported.
Nuclear localization signals and nuclear export signals on proteins to be transported through NPCs are recognized by corresponding nuclear transport receptors. These receptors function by binding their cargoes selectively on one side of the nuclear envelope, increasing the diffusion rate through NPCs, and releasing cargoes selectively on the other side. The free energy of GTP hydrolysis by the monomeric GTPase Ran is harnessed to provide the directionality for nuclear transport. Messenger RNAs are exported from the nucleus through NPCs as parts of large ribonucleoprotein complexes; they use a different transport route that uses ATP hydrolysis to remodel the complexes at the cytosolic side of NPCs. Cells regulate the transport of nuclear proteins and RNA molecules through the NPCs by controlling the access of these molecules to the transport machinery. Because nuclear localization signals are not removed, nuclear proteins can be imported repeatedly, as is required each time that the nucleus reassembles after mitosis.
Glossary
- nuclear envelope
- The double membrane (two bilayers) surrounding the nucleus. Consists of an outer and inner membrane and is perforated by nuclear pores. The outer membrane is continuous with the endoplasmic reticulum.
- inner nuclear membrane
- One of two concentric membranes comprising the nuclear envelope; contains specific proteins as anchoring sites for chromatin and the nuclear lamina.
- nuclear lamina
- Fibrous meshwork of proteins on the inner surface of the inner nuclear membrane. It is made up of a network of intermediate filaments formed from nuclear lamins.
- outer nuclear membrane
- One of two concentric membranes comprising the nuclear envelope; surrounds the inner nuclear membrane and is continuous with the inner nuclear membrane and the membrane of the endoplasmic reticulum.
- nuclear pore complexes (NPCs) (NPC)
- Large multiprotein structure forming an aqueous channel (the nuclear pore) through the nuclear envelope; it allows selected molecules to move between nucleus and cytoplasm.
- nucleoporins
- Any of a number of different proteins that make up nuclear pore complexes.
- nuclear localization signals (NLSs) (NLS)
- Signal sequence or signal patch found in proteins destined for the nucleus that enables their selective transport into the nucleus from the cytosol through the nuclear pore complexes.
- nuclear transport receptors (karyopherin)
- Protein that escorts macromolecules either into or out of the nucleus: nuclear import receptor or nuclear export receptor.
- nuclear import receptors
- Proteins that recognize nuclear localization signals to initiate the nuclear import of proteins containing the appropriate nuclear localization signal.
- nuclear export receptors
- Proteins that bind to both the export signal and nuclear pore complex proteins, so as to guide their cargo through the nuclear pore complex to the cytosol.
- Ran (Ran protein)
- Monomeric GTPase of the Ras superfamily present in both cytosol and nucleus. It is required for the active transport of macromolecules into and out of the nucleus through nuclear pore complexes.
- nuclear export signals
- Sorting signal contained in the structure of those protein molecules and RNA–protein complexes that are to be transported from the nucleus to the cytosol through nuclear pore complexes; includes nuclear RNPs and new ribosomal subunits.
- nuclear lamin
- Protein subunit of the intermediate filaments that form the nuclear lamina.