Chapter 1Elements of the Immune System and Their Roles in Defense
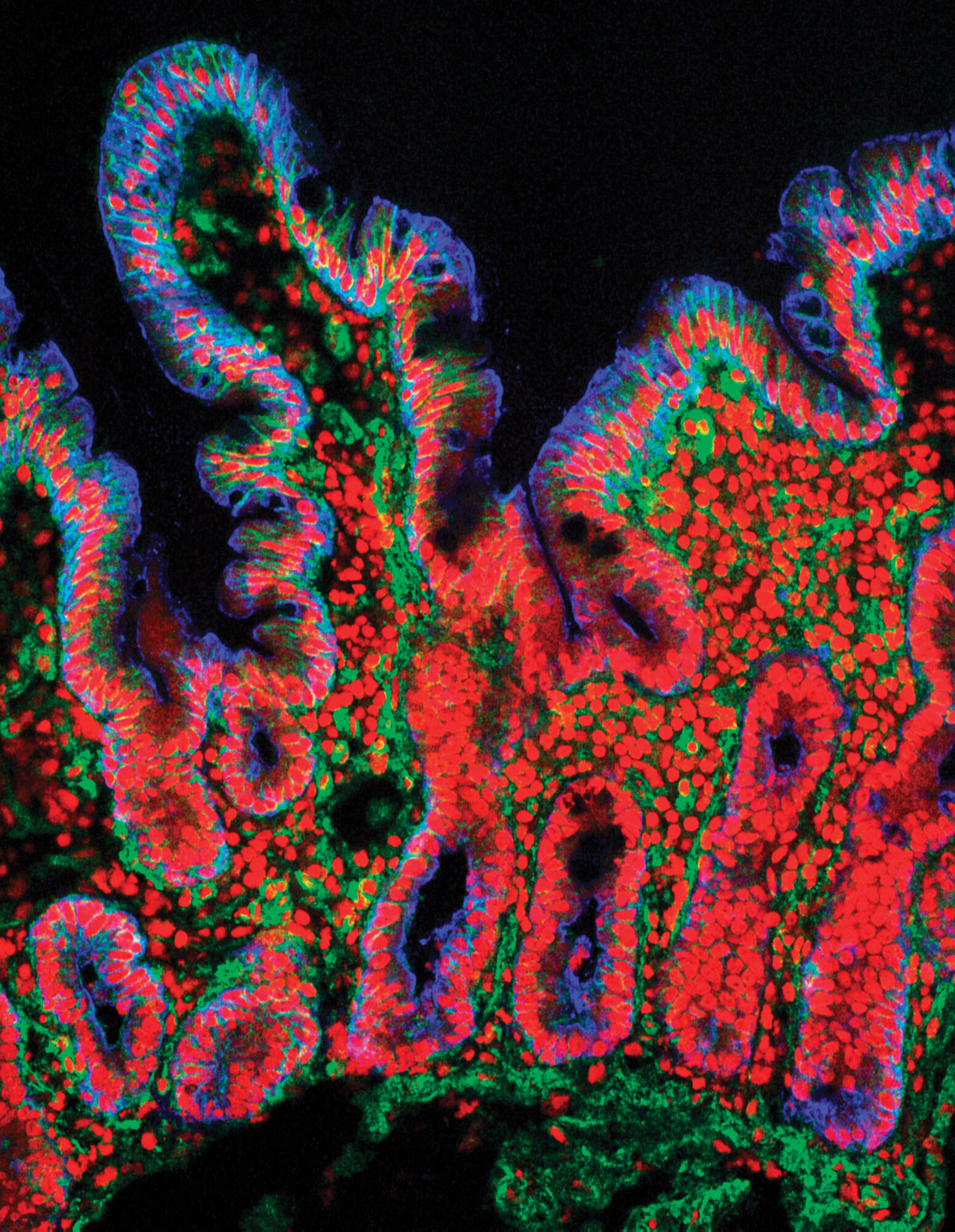
Immunology is the study of the physiological mechanisms that humans and other mammals use to defend their bodies from invasion by other organisms. The origins of the subject lie in the practice of medicine, with historical observations that people who survived an epidemic disease were untouched when faced with that disease again—they had become immune to infection. Infectious diseases are caused by microorganisms, which reproduce and evolve more rapidly than their human hosts. During an infection, the microorganism pits a vast population of its species against an individual Homo sapiens. In response, the human body invests heavily in cells that are dedicated to defense and collectively form the immune system.
An immune system is crucial to human survival. In the absence of a working immune system, even minor infections take hold and prove fatal. Without intensive treatment, children born without an immune system die in early childhood from common infections. However, all humans suffer infectious diseases, especially when young. To provide an immunity that gives long-lasting future protection, the immune system must first do battle with the causative microorganism. This places children at highest risk during their first infection with a microorganism and, without modern medicine, leads to substantial child mortality, as occurs in the developing world. When entire populations face a new infection, the outcome can be catastrophic, as experienced by indigenous peoples who were killed in large numbers by European diseases that were imported with the arrival of Europeans in the Americas in 1492 and the subsequent initiation of transatlantic trade.
New diseases are still emerging. The coronavirus SARS-CoV-2, which causes the respiratory disease COVID-19, was first detected in Wuhan, China, in December 2019, although the virus is thought to have been circulating for a few months before that, according to both retrospective testing of earlier samples collected for other purposes and variations in the genome sequences of viruses from different samples. Within a few weeks, infected individuals had carried the virus around the globe along the modern trading routes of the jet airliner and cruise ship, resulting in a pandemic. By early September 2020, more than 27 million people had been infected with SARS-CoV-2 and nearly 900,000 of them had perished. In many countries, normal life and commerce ground to a standstill as people stayed at home to reduce the spread of the virus.
In medicine, the greatest triumph of immunology is vaccination, a procedure that prevents severe disease by exposing healthy infants to the infectious agent in a form that does not cause disease. Vaccination provides human immune systems with the experience they need to make a strong response, but one with little risk to health and life. Vaccination was first used against smallpox, a viral scourge that for centuries decimated human populations and disfigured the faces of the survivors. In Asia, a small dose of smallpox virus was used to induce immunity long before 1721, when Lady Mary Wortley Montagu introduced the method to Europeans. In 1796, Edward Jenner, a doctor in rural England, showed how inoculation with cowpox virus protected against the related smallpox virus, but with less risk of causing disease than the earlier method. Jenner called his procedure vaccination, vaccinia being the name of the mild disease caused by cowpox. Since Jenner’s time, vaccination progressively reduced the incidence of smallpox worldwide, until it was eventually eliminated in the 1970s (Figure 1.1).
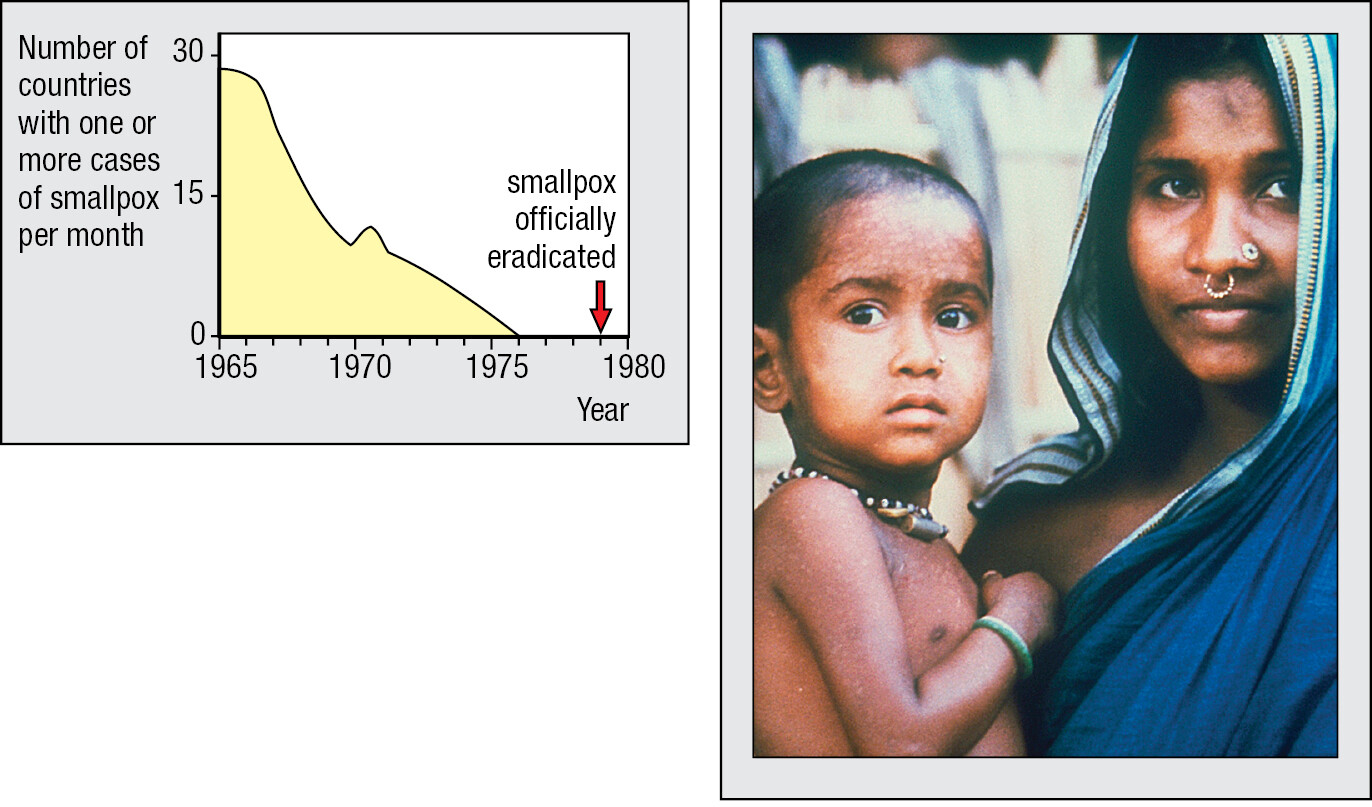
Effective vaccines have been made for only a fraction of the infectious diseases, and for some vaccines the application has been limited by cost. The widely used vaccines were developed by trial and error, in an era when little was known of the immune system’s components and how they work. That approach is no longer successful in vaccine development; all the easily won vaccines seem to have been made. Today’s greater knowledge and deeper understanding of the mechanisms of immunity are spawning new ideas for vaccines against infectious diseases, and also for noninfectious diseases such as cancer. All these new ideas and technology are being applied to the development and manufacture of vaccines and therapeutics for the purpose of ending the SARS-CoV-2 pandemic. To that end, 52,000 papers on SARS-CoV-2 were published in the biomedical journals in the 6 months from January to June 2020, and many more have been published since then. The casualties of SARS-CoV-2 include vaccination programs in 23 African countries that were suspended because of the chaos. In 2020, 13.5 million children missed out on vaccinations against polio, measles, human papilloma virus, yellow fever, cholera, and meningitis.
In this chapter, we consider the microorganisms that infect human beings and the defenses the microorganisms must overcome to start and propagate an infection. The individual cells and tissues of the immune system and how they integrate with the rest of the human body are described. The first line of defense is innate immunity, which combines physical and chemical barriers with rapid responses that eliminate infecting microorganisms before they disrupt human tissue. These mechanisms stop most infections, but when they fail, the targeted and forceful defenses of adaptive immunity are brought into play. These adapt to the particular microorganism and are continually refined in the course of infection. Usually successful, the adaptive immune response clears the infection and has a long-lasting memory that prevents its recurrence.
1-1Numerous commensal microorganisms inhabit healthy human bodies
The remit of the immune system is to protect the human body from infectious disease. Most infectious diseases of humans are caused by microorganisms, which are smaller than a human cell. For both benign and dangerous microorganisms, the human body constitutes an extensive resource-rich environment in which to feed, live, and reproduce. More than 1000 different microbial species dwell in a healthy adult human’s gut, and they constitute about 4.5 kg of the body’s weight. They are called commensal microorganisms because they ‘eat at the same table’ as their human host. The entire community of microbial species that inhabits the human body—skin, mouth, gut, or vagina—is called the microbiota. Different ecological niches within the body have distinctive microbiota and are described as the ‘gut microbiota,’ the ‘oral microbiota,’ and so on. The biology of many commensal species has yet to be studied in any depth because they cannot yet be grown in the laboratory. We know they are there, because their distinctive nucleic acid sequences were discovered from analyses of human feces.
Animals coevolve with their commensal species and become both tolerant of them and dependent on them. Commensal organisms enhance human nutrition by processing digested food and making some of our essential vitamins. They also protect against disease, because their presence helps to prevent colonization by disease-causing microorganisms. For example, as well as competing for space, the bacterium Escherichia coli, a major component of the healthy human gut microbiota, secretes antibacterial proteins called colicins that incapacitate other bacterial species and prevent their colonization of the human gut. When a person with a bacterial infection is treated with antibiotics, much of the gut microbiota is killed along with the disease-causing bacteria. After such treatment, the body recolonizes with a new population of commensal bacteria, a situation in which opportunistic bacteria, such as Clostridium difficile, can become established and cause disease. Clostridium difficile is present in small numbers in healthy individuals, but in patients treated with antibiotics it can proliferate (Figure 1.2). It secretes a toxin that inflames the colon. This causes diarrhea and bleeding and can lead to the serious and potentially fatal condition of pseudomembranous colitis.
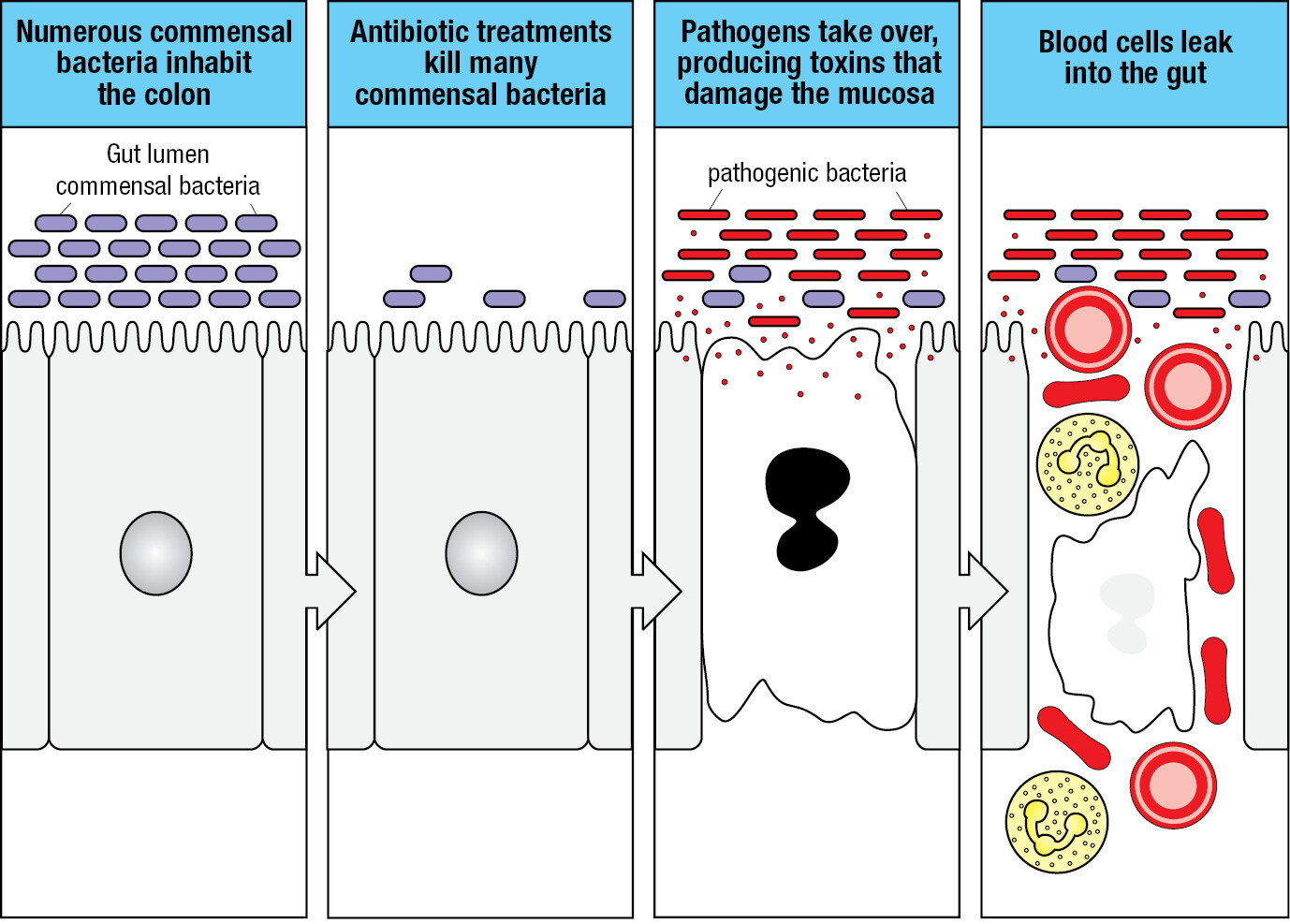
1-2Pathogens are infectious organisms that cause disease
Any microorganism that causes disease is termed a pathogen. This definition includes microbes such as the influenza virus and the typhoid bacillus that habitually cause disease, and also microorganisms that are usually harmless but cause disease if a person’s immune system and other defenses of the body are weakened. The latter category comprises the opportunistic pathogens. In the early years of the human immunodeficiency virus (HIV) pandemic, when there was no effective therapy, almost all people who became infected died. Their immune systems became worn out by the HIV infection, but their deaths were caused by various opportunistic pathogens.
Pathogens are of four kinds. The viruses, bacteria, and fungi are all groups of related microorganisms. The internal parasites are a heterogeneous group of unicellular protozoa and multicellular invertebrates, mainly worms. In this book we consider the functions of the human immune system principally in the context of controlling infection. For some pathogens this necessitates their complete elimination, but for others it is sufficient to limit the size of the pathogen population and its anatomical location within the human host. Figure 1.3 illustrates the variety in shape and form of the four kinds of pathogen. Figure 1.4 lists common or well-known infectious diseases and the pathogens that cause them. Reference to many of these diseases and the problems they pose for the immune system are made throughout this book.
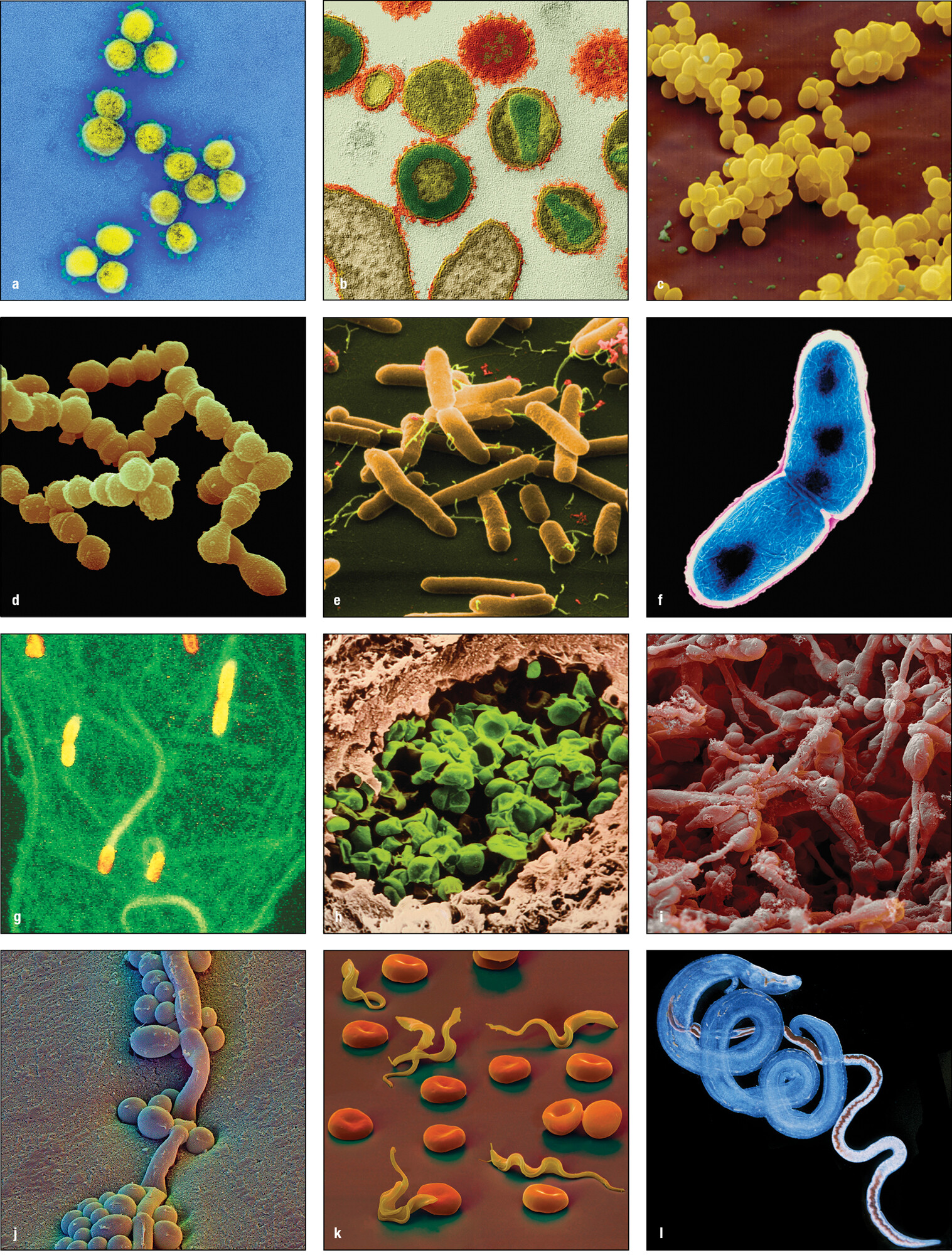
Type |
Disease |
Pathogen |
General classification* |
Route of infection |
---|---|---|---|---|
Viruses |
||||
Coronavirus disease 2019 (COVID-19) |
SARS-CoV-2 |
Coronaviruses |
Oral/respiratory/ocular mucosa |
|
Severe acute respiratory syndrome |
SARS-CoV |
Coronaviruses |
Oral/respiratory/ocular mucosa |
|
West Nile encephalitis |
West Nile virus |
Flaviviruses |
Bite of an infected mosquito |
|
Yellow fever |
Yellow fever virus |
Flaviviruses |
Bite of infected mosquito (Aedes aegypti) |
|
Hepatitis B |
Hepatitis B virus |
Hepadnaviruses |
Sexual transmission; infected blood |
|
Chickenpox |
Varicella-zoster |
Herpesviruses |
Oral/respiratory |
|
Mononucleosis |
Epstein–Barr virus |
Herpesviruses |
Oral/respiratory |
|
Influenza |
Influenza virus |
Orthomyxoviruses |
Oral/respiratory |
|
Measles |
Measles virus |
Paramyxoviruses |
Oral/respiratory |
|
Mumps |
Mumps virus |
Paramyxoviruses |
Oral/respiratory |
|
Poliomyelitis |
Polio virus |
Picornaviruses |
Oral |
|
Jaundice |
Hepatitis A virus |
Picornaviruses |
Oral |
|
Smallpox |
Variola |
Poxviruses |
Oral/respiratory |
|
AIDS |
Human immunodeficiency virus |
Retroviruses |
Sexual transmission, infected blood |
|
Rabies |
Rabies virus |
Rhabdoviruses |
Bite of an infected animal |
|
Common cold |
Rhinoviruses |
Rhinoviruses |
Nasal |
|
Diarrhea |
Rotavirus |
Rotaviruses |
Oral |
|
Rubella |
Rubella |
Togaviruses |
Oral/respiratory |
|
Bacteria |
Trachoma |
Chlamydia trachomatis |
Chlamydias |
Oral/respiratory/ocular mucosa |
Bacillary dysentery |
Shigella flexneri |
Gram-negative bacilli |
Oral |
|
Food poisoning |
Salmonella enterica serovar Enteritidis, S. Typhimurium |
Gram-negative bacilli |
Oral |
|
Plague |
Yersinia pestis |
Gram-negative bacilli |
Infected flea bite, respiratory |
|
Tularemia |
Francisella tularensis |
Gram-negative bacilli |
Handling infected animals |
|
Typhoid fever |
Salmonella Typhi |
Gram-negative bacilli |
Oral |
|
Gonorrhea |
Neisseria gonorrhoeae |
Gram-negative cocci |
Sexually transmitted |
|
Meningococcal meningitis |
Neisseria meningitidis |
Gram-negative cocci |
Oral/respiratory |
|
Meningitis, pneumonia |
Haemophilus influenzae |
Gram-negative coccobacilli |
Oral/respiratory |
|
Legionnaire’s disease |
Legionella pneumophila |
Gram-negative coccobacilli |
Inhalation of contaminated aerosol |
|
Whooping cough |
Bordetella pertussis |
Gram-negative coccobacilli |
Oral/respiratory |
|
Cholera |
Vibrio cholerae |
Gram-negative vibrios |
Oral |
|
Anthrax |
Bacillus anthracis |
Gram-positive bacilli |
Oral/respiratory by contact with spores |
|
Diphtheria |
Corynebacterium diphtheriae |
Gram-positive bacilli |
Oral/respiratory |
|
Tetanus |
Clostridium tetani |
Gram-positive bacilli (anaerobic) |
Infected wound |
|
Boils, wound infections |
Staphylococcus aureus |
Gram-positive cocci |
Wounds; oral/respiratory |
|
Pneumonia, scarlet fever |
Streptococcus pneumoniae |
Gram-positive cocci |
Oral/respiratory |
|
Tonsillitis |
Streptococcus pyogenes |
Gram-positive cocci |
Oral/respiratory |
|
Leprosy |
Mycobacterium leprae |
Mycobacteria |
Infected respiratory droplets |
|
Tuberculosis |
Mycobacterium tuberculosis |
Mycobacteria |
Oral/respiratory |
|
Respiratory disease |
Mycoplasma pneumoniae |
Mycoplasmas |
Oral/respiratory |
|
Typhus |
Rickettsia prowazekii |
Rickettsias |
Bite of infected tick |
|
Lyme disease |
Borrelia burgdorferi |
Spirochetes |
Bite of infected deer tick |
|
Syphilis |
Treponema pallidum |
Spirochetes |
Sexual transmission |
|
Fungi |
Aspergillosis |
Aspergillus species |
Ascomycetes |
Opportunistic pathogen, inhalation of spores |
Athlete’s foot |
Trichophyton species |
Ascomycetes |
Physical contact |
|
Candidiasis, thrush |
Candida albicans |
Ascomycetes (yeasts) |
Opportunistic pathogen, resident microbiota |
|
Pneumonia |
Pneumocystis jirovecii |
Ascomycetes |
Opportunistic pathogen, resident lung microbiota |
|
Protozoan parasites |
Leishmaniasis |
Leishmania major |
Protozoa |
Bite of an infected sand fly |
Malaria |
Plasmodium falciparum |
Protozoa |
Bite of an infected mosquito |
|
Toxoplasmosis |
Toxoplasma gondii |
Protozoa |
Oral, from infected material |
|
Trypanosomiasis |
Trypanosoma brucei |
Protozoa |
Bite of an infected tsetse fly |
|
Helminth parasites (worms) |
Common roundworm |
Ascaris lumbricoides |
Nematodes (roundworms) |
Oral, from infected material |
Schistosomiasis |
Schistosoma mansoni |
Trematodes |
Through skin by bathing in infected water |
Over evolutionary time, the relationship between a pathogen and its human host can change in ways that affect disease severity. Pathogenic organisms evolve adaptations that enable them to invade their hosts, replicate within them, and be propagated through a human population. Rapid death of the human host is rarely in a microbe’s interest, because this destroys its home and source of sustenance. Consequently, those organisms with potential to inflict severe and fatal disease often evolve an accommodation with their host. In complementary fashion, human populations evolve a genetic resistance to common disease-causing organisms; the diseases they cause are known as endemic diseases. These diseases, such as measles, chickenpox, and malaria, are ubiquitous in a given population, with most people being exposed during childhood. Because of the interplay between host and pathogen, the nature and severity of infectious diseases in human populations are always changing.
Influenza is an example of a common viral disease that has severe symptoms but is usually overcome by the human immune system. The fever, aches, and lassitude that accompany infection can seem overwhelming, and it is difficult to imagine overcoming foes or predators at the peak of a bout of influenza. Nevertheless, despite the severity of the symptoms, most strains of influenza pose no great danger to healthy people in populations where influenza is endemic. Warm, well-nourished, and otherwise healthy people usually recover within 2 weeks and assume that their immune system will accomplish this task. Contrasting with influenza is Ebola virus, a pathogen new to human populations and which causes a high mortality of 60–75% among those infected. The effects of SARS-CoV-2 on human populations are less severe than those of Ebola but much greater than those of seasonal influenza and the common colds caused by other strains of coronavirus. COVID-19 is killing large numbers of people, but most of them were vulnerable because of other, preexisting conditions.
1-3Skin and mucosal surfaces are barrier defenses against infection
The skin gives the human body a formidable defense against infection. It is an epithelium protected by a tough, impenetrable outer barrier of layers of keratinized cells. Epithelium is a general name for the layers of cells that line the outer surface and the inner cavities of the body. However, the skin can be breached by physical damage, such as wounds, burns, or surgical procedures, which exposes soft tissues and renders them vulnerable to infection. Until the adoption of antiseptic procedures in the 19th century, surgery was a risky business, largely because of the life-threatening infections that the procedures introduced. Consequently, far more soldiers died from infected wounds than from the direct effects of enemy action. Ironically, the need to conduct increasingly sophisticated and wide-ranging warfare has been the major force driving improvements in surgery and medicine. As an example from immunology, the horrific burns suffered by fighter pilots during the Second World War stimulated the study and application of skin transplantation, which directly led to knowledge of the cellular basis of the immune response.
Continuous with the skin are the epithelia that line the respiratory, gastrointestinal, and urogenital tracts (Figure 1.5). On these internal surfaces, the impermeable skin gives way to specialized tissues that communicate with the environment and are vulnerable to microbial invasion. These tissues, the mucosal surfaces, or mucosae, are coated with the mucus they constitutively secrete. This thick fluid layer contains glycoproteins, proteoglycans, and enzymes that protect the epithelial cells from damage and contribute to limiting infection. In the respiratory tract, mucus is continually removed through the beating action of the cilia that characterize this epithelium. The mucus bathing the epithelium is replenished by goblet cells, specialized in the synthesis and secretion of mucus. In this way the respiratory mucosa is continually cleansed of unwanted material, particularly infectious microorganisms.
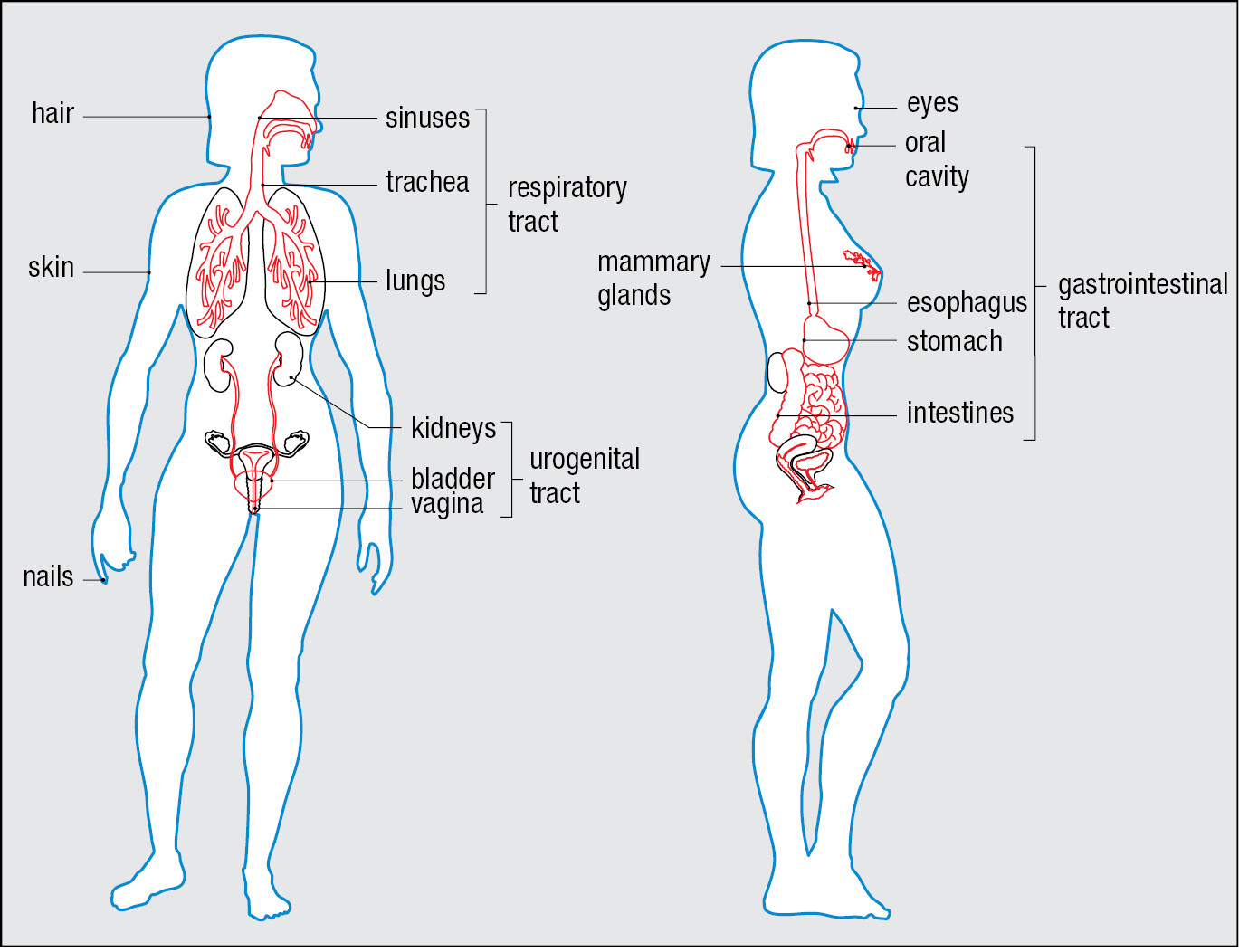
Epithelial surfaces also secrete antimicrobial substances. Sebum secreted by the sebaceous glands associated with hair follicles contains fatty acids and lactic acids, which cooperate to inhibit bacterial growth at the skin surface. All epithelia produce antimicrobial peptides that kill bacteria, fungi, and enveloped viruses by the common mechanism of perturbing their membranes. Tears and saliva contain lysozyme, an enzyme that kills bacteria by degrading their cell walls. Also deterring microorganisms are the acidic environments of the stomach, vagina, and skin.
The fixed defenses of skin and mucosa provide well-maintained mechanical, chemical, and microbiological barriers that prevent most pathogens from gaining access to the cells and tissues of the body. When those barriers are breached and pathogens gain entry to the body’s soft tissues, the defense mechanisms of innate immunity are aimed at the invaders.
1-4The innate immune response produces a state of inflammation at sites of infection
Cuts, abrasions, bites, and wounds provide routes for pathogens to get through the skin. Touching, rubbing, picking, and poking the eyes, nose, and mouth help pathogens breach mucosal surfaces, as does breathing polluted air, eating contaminated food, and being around infected people. With few exceptions, the infections remain localized and are extinguished within a few days without incapacitation or illness. Such infections are controlled and terminated by the innate immune response, which is always ready to react. The response consists of two phases (Figure 1.6). The first is recognition that a pathogen is present. This involves soluble proteins and cell-surface receptors that bind either to the pathogen or to human cells and plasma proteins that have been altered by the pathogen’s presence. Once the pathogen has been recognized, the second phase of the response recruits effector mechanisms that kill or eliminate the pathogen. Mediating the effector mechanisms are effector cells that engulf bacteria, kill virus-infected cells, and attack protozoa. Guiding the effector cells is complement, a system of plasma proteins that commands the effector cells by tagging pathogens with molecular flags. Complement proteins can also kill pathogens without assistance from effector cells by perturbing the integrity of the pathogens’ membranes. Collectively, these defenses comprise innate immunity, a genetically programmed set of responses that can act immediately when an infection occurs. Numerous families of receptor proteins contribute to pathogen recognition in the innate immune response. They are of many different structural types and have binding specificities for chemically diverse ligands. These ligands include peptides, proteins, glycoproteins, proteoglycans, peptidoglycans, carbohydrates, glycolipids, phospholipids, nucleic acids, small molecules, and metabolites.
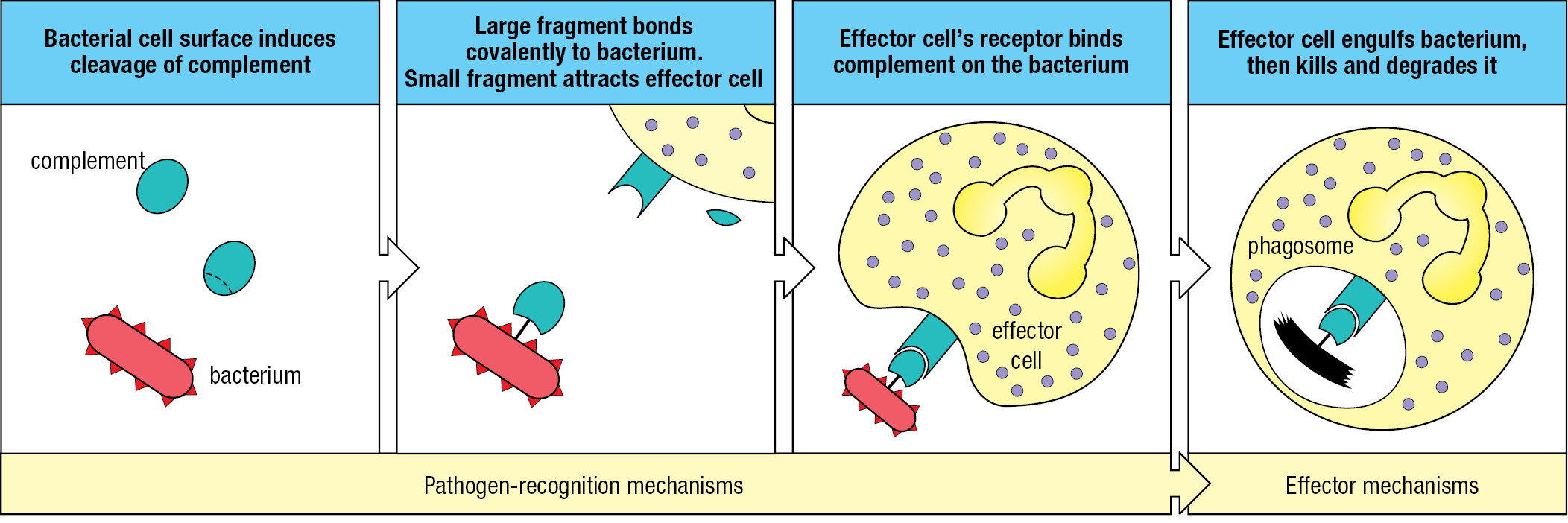
During play and exploration of the local environment, small wounds to the skin can be a daily occurrence for many children. On returning home, the grazes are washed, which removes most of the dirt and the bacteria associated with soil, plants, and wild and domesticated animals, including other humans. Of the bacteria that remain, some divide and start an infection. The receptors of cells in the damaged tissue detect the bacteria, and the cells send out small messenger proteins called cytokines that bind receptors on the effector cells of innate immunity to trigger the innate immune response. The overall effect of the innate immune response is to induce a state of inflammation in the infected tissue. An ancient concept in medicine, inflammation was defined by calor, dolor, rubor, and tumor: the Latin words for heat, pain, redness, and swelling. These symptoms are not caused by the infecting pathogen but by the human innate immune response to the pathogen.
Cytokines induce dilation of nearby blood capillaries, which increases the blood flow, causing the skin to warm and redden. Vascular dilation (vasodilation) creates gaps between the cells of the endothelium, the thin layer of specialized epithelium that lines the interior of blood vessels. This makes the endothelium more permeable, increasing the leakage of blood plasma into connective tissue. Expansion of the local fluid volume, or edema, causes swelling, putting pressure on nerve endings and causing pain. Cytokines alter the adhesive properties of the vascular endothelium, permitting white blood cells to leave the blood and enter the inflamed tissue (Figure 1.7). White blood cells recruited to the infected tissue contribute to the inflammation and in this context are called inflammatory cells. Infiltration of cells into the inflamed tissue increases the swelling, and the cells release chemicals that cause pain. The upside of the discomfort and disfigurement caused by inflammation is that it enables an army of immune-system cells and soluble effector molecules to be brought rapidly and in quantity to the infected tissue.
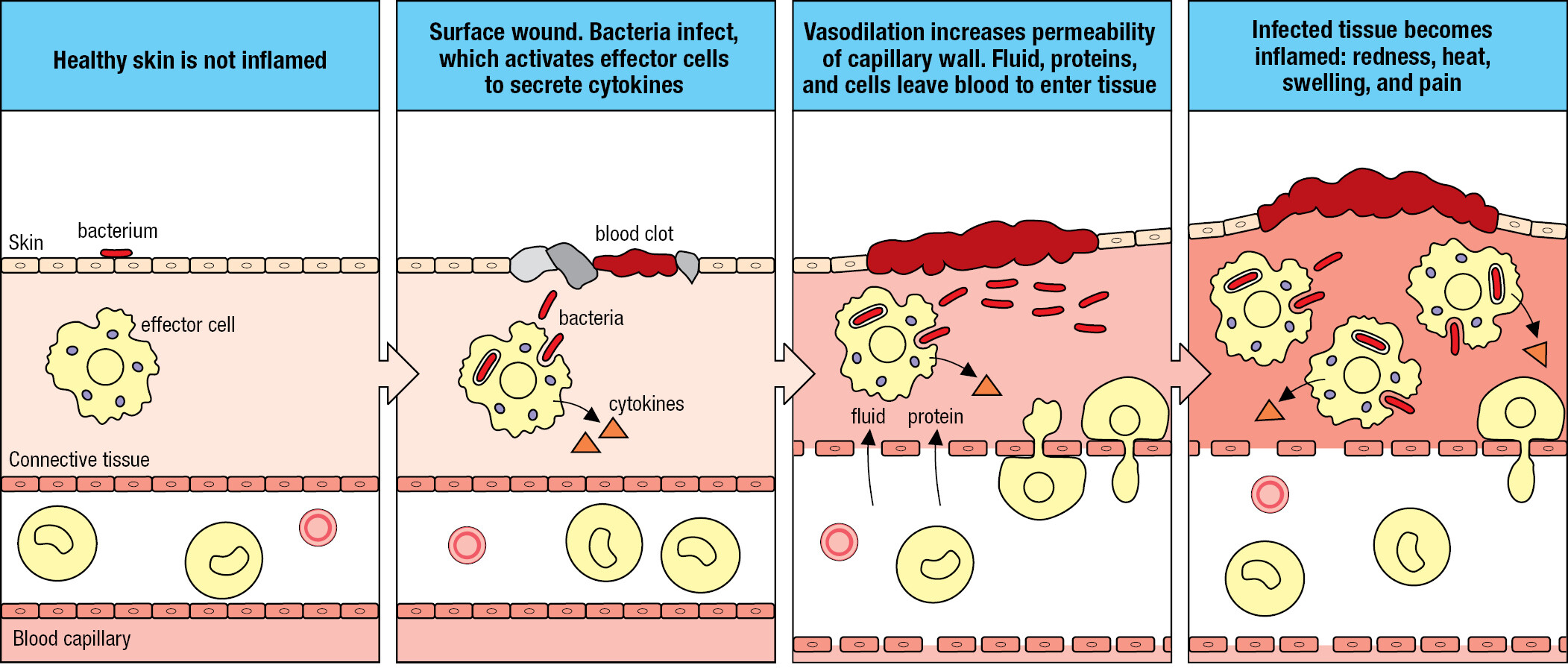
Historically, the study of immunology (adaptive immunity) and the study of inflammation (innate immunity) were separate medical disciplines, and they began to merge only in the 1980s. Today, the terms inflammation and innate immunity are used interchangeably. The mechanisms of innate immunity are considered in Chapters 2 and 3.
1-5The adaptive immune response builds on the innate immune response
Everybody is exposed to pathogens every day. The intensity of exposure and the diversity of the encountered pathogens increase with crowded city living and the daily exchange of people and pathogens in international airports and other transport hubs. Despite the exposure, innate immunity keeps most people healthy for most of the time. Nevertheless, some infections outrun the innate immune response, an event more frequent in people who are poor, malnourished, badly housed, deprived of sleep, or stressed and insecure in other ways. When this occurs, the innate immune response works to minimize the spread of infection while enlisting white blood cells called lymphocytes to increase the strength and focus of the immune response. The unique contribution that lymphocytes make to the defense of the human body is the adaptive immune response. This is organized around the existing infection and the innate immune response and adapts that response to the unique characteristics of the infecting pathogen. Consequently, the adaptive immunity that is directed at the pathogen is powerful, long lasting, and specific to that pathogen. Adaptive immunity has evolved only in vertebrate animals, where it complements the mechanisms of innate immunity that vertebrates and invertebrates have in common.
The effector mechanisms of adaptive immunity are largely those used in innate immunity. The important differences between innate immunity and adaptive immunity are in the receptors that lymphocytes use to recognize pathogens (Figure 1.8). The receptors of innate immunity are structurally of many different types. Each receptor recognizes molecular features shared by groups of pathogens, and none is specific for a particular pathogen. Conversely, the lymphocytes of adaptive immunity recognize pathogens using only one type of cell-surface receptor, but this is made in billions of different versions. The adaptive immune response becomes specific for a particular pathogen by including only lymphocytes expressing a receptor that recognizes the pathogen. Lymphocyte receptors are not encoded by conventional genes, but by genes that are cut, spliced, and modified during lymphocyte development. By these mechanisms, each lymphocyte is programmed to make one variant of the basic receptor type. Billions of different receptor variants are represented in the lymphocytes of a person’s immune system, which enables all possible pathogens to be recognized by the immune system.
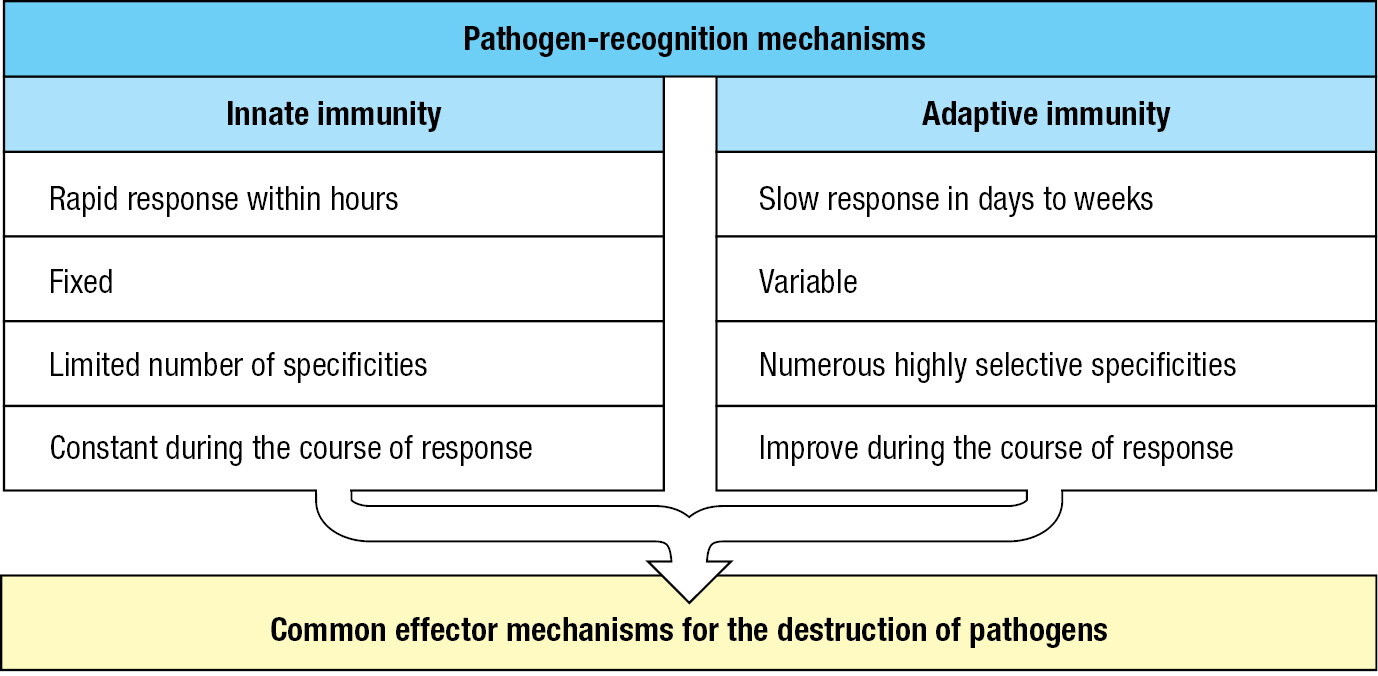
During infection, only lymphocytes bearing receptors that recognize the infecting pathogen are recruited to the adaptive immune response. They then proliferate and differentiate to form an army of pathogen-specific effector cells (Figure 1.9). The processes that select pathogen-specific lymphocytes for proliferation and differentiation are called clonal selection and clonal expansion. Because of the time it takes to expand a few selected lymphocytes into an army, the benefit of the adaptive immune response cannot be felt until 7–10 days after the onset of infection.
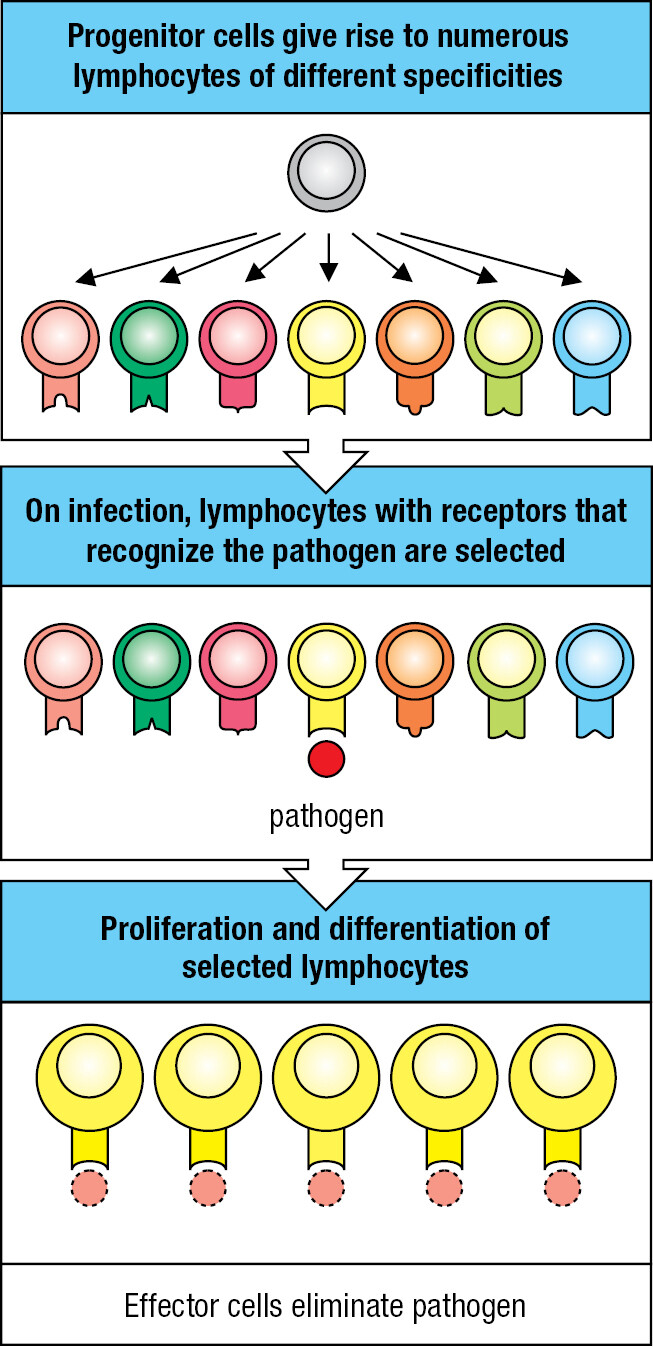
The ‘flu’ is a global disease that most people have experienced. It starts when epithelial cells in the lower respiratory tract become infected with influenza virus. The debilitating symptoms emerge 3 or 4 days after the start of infection, when the spread of the virus outruns the innate immune response. The disease persists for 5–7 days while an adaptive immune response is developed and then put to work. As the adaptive immune response gains the upper hand, fever subsides and a gradual convalescence begins in the second week after infection.
Some of the lymphocytes that contributed to a successful adaptive immune response persist in the body and are selected to provide long-term immunological memory of the pathogen. These memory cells enable subsequent encounters with the same pathogen to elicit a stronger and faster adaptive immune response; one that terminates infection before there are any significant symptoms of disease. The adaptive immunity based on immunological memory is also called acquired immunity, or protective immunity. For some pathogens, such as measles virus, one full-blown infection provides immunity for decades, whereas for influenza virus the protection is less effective. This is not due to faulty memory but to the rapid evolution of the virus, which allows it to escape the immunity acquired by its human hosts.
The first time that a person makes an adaptive immune response to a pathogen is called the primary immune response. During the primary response, the person acquires the immunological memory that enables subsequent encounters with the pathogen to be met with a faster and stronger secondary immune response. The purpose of vaccination is to provide people with a good immunological memory of the pathogen without them having to experience the disease. To do this, any vaccine must stimulate strong innate and adaptive immune responses against the pathogen (Figure 1.10). Immunological memory and vaccination are considered in Chapter 11.
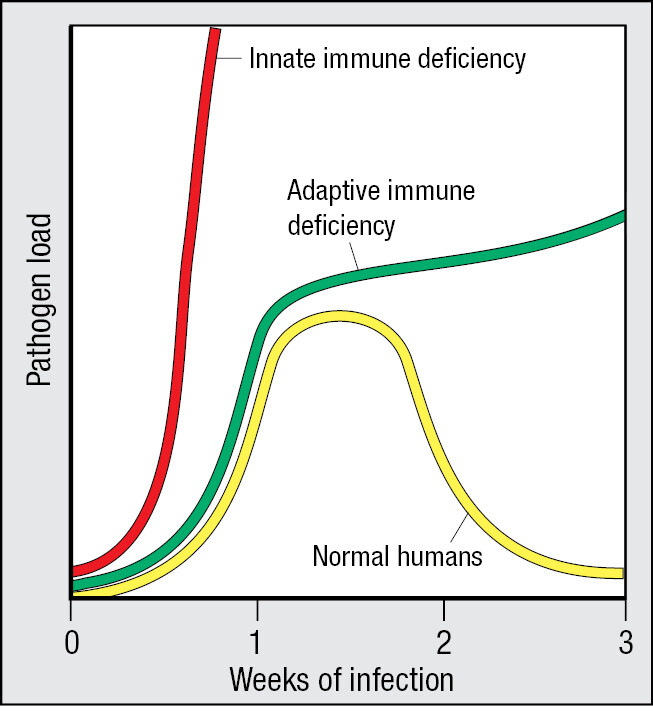
1-6Immune-system cells with different functions derive from hematopoietic stem cells
The cells of the immune system are principally the white blood cells, or leukocytes, and tissue cells related to them. They and other blood cells are continually being generated in the body by the process of hematopoiesis. Leukocytes derive from a common progenitor called the pluripotent hematopoietic stem cell, which also gives rise to red blood cells (erythrocytes) and megakaryocytes, the source of platelets. These cells and their developmental precursors are collectively called hematopoietic cells (Figure 1.11). The anatomical site for hematopoiesis changes with age (Figure 1.12). In the early embryo, blood cells are first produced in the yolk sac and later in the fetal liver. From the third to the seventh month of fetal life, the spleen becomes the major site of hematopoiesis. As the bones develop during the fourth and fifth months of fetal growth, hematopoiesis begins to shift to the bone marrow, and by the time of birth practically all hematopoiesis takes place there. In adults, hematopoiesis is concentrated in the marrow of the skull, ribs, sternum, vertebral column, pelvis, and femurs. As blood cells are short-lived, they must continually be renewed, so hematopoiesis remains active throughout life.
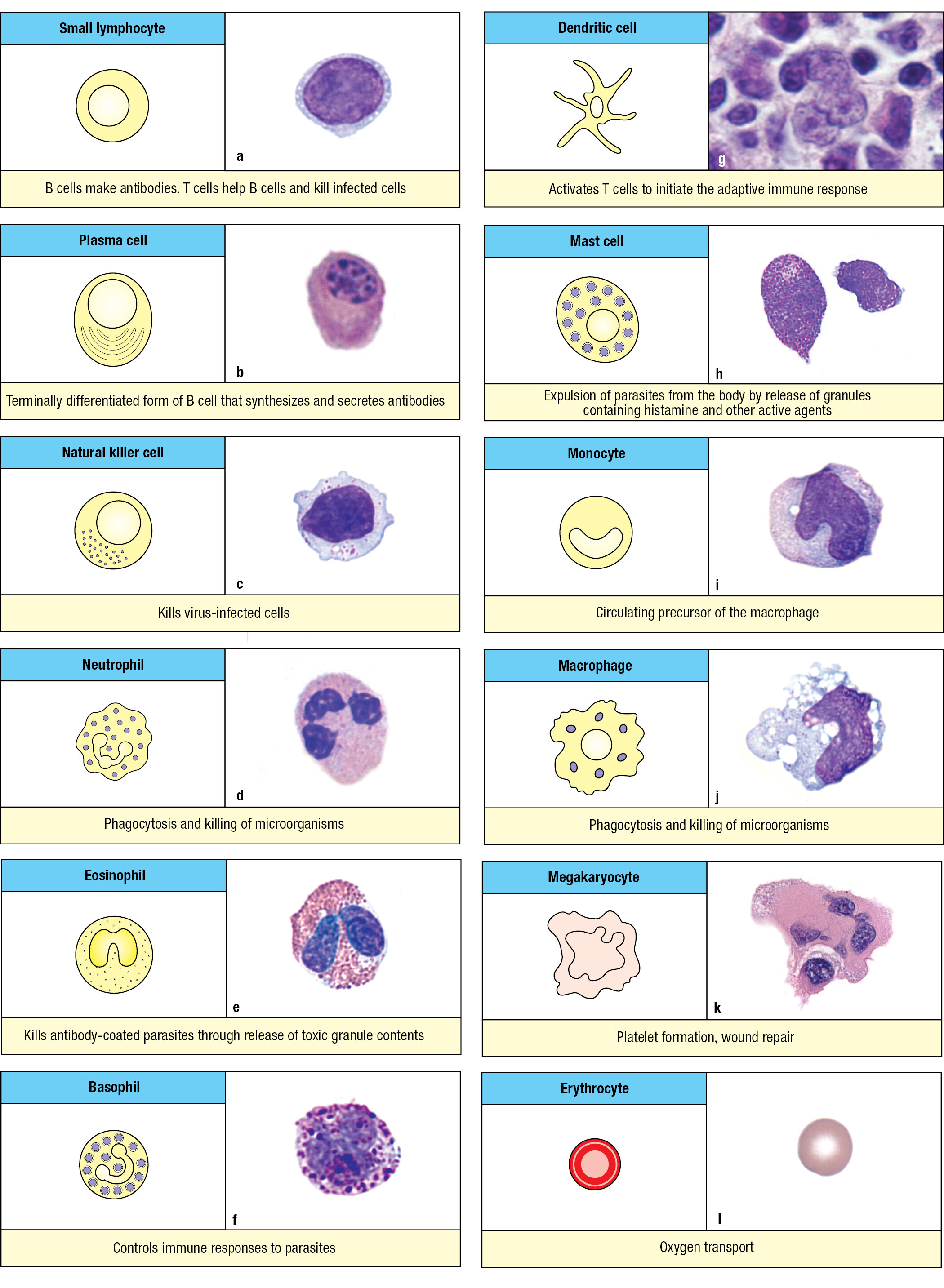
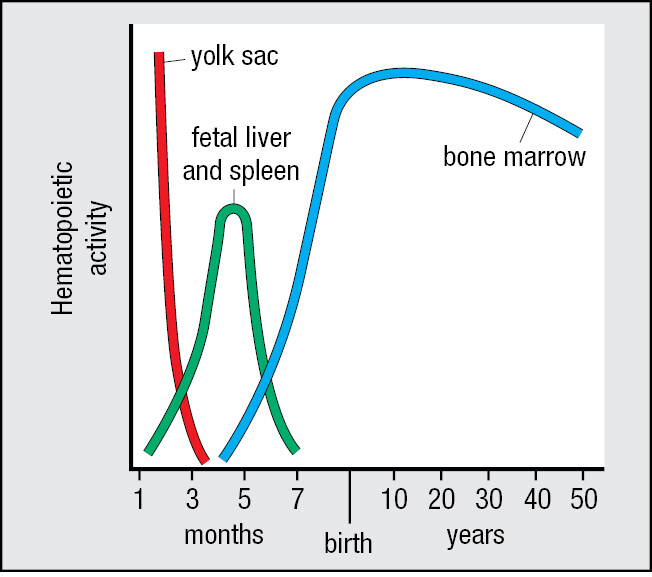
Hematopoietic stem cells divide to give daughter cells that are also hematopoietic stem cells, a process of self renewal. Alternatively, daughter cells can differentiate to become more mature stem cells, which commit to one of three cell lineages: the erythroid, myeloid, and lymphoid lineages (Figure 1.13). The megakaryocyte–erythroid precursor cell gives rise to the oxygen-carrying erythrocytes and the platelet-producing megakaryocytes. Megakaryocytes are giant cells that arise from the fusion of multiple precursor cells and have nuclei containing multiple sets of chromosomes (megakaryocyte means ‘cell with giant nucleus’). Megakaryocytes permanently reside in the bone marrow. Platelets are small plate-like packets of membrane-enclosed cytoplasm that break off from a megakaryocyte. Their function is to maintain the integrity of blood vessels. Platelets initiate and participate in the clotting reactions that close off badly damaged blood vessels to reduce blood loss.
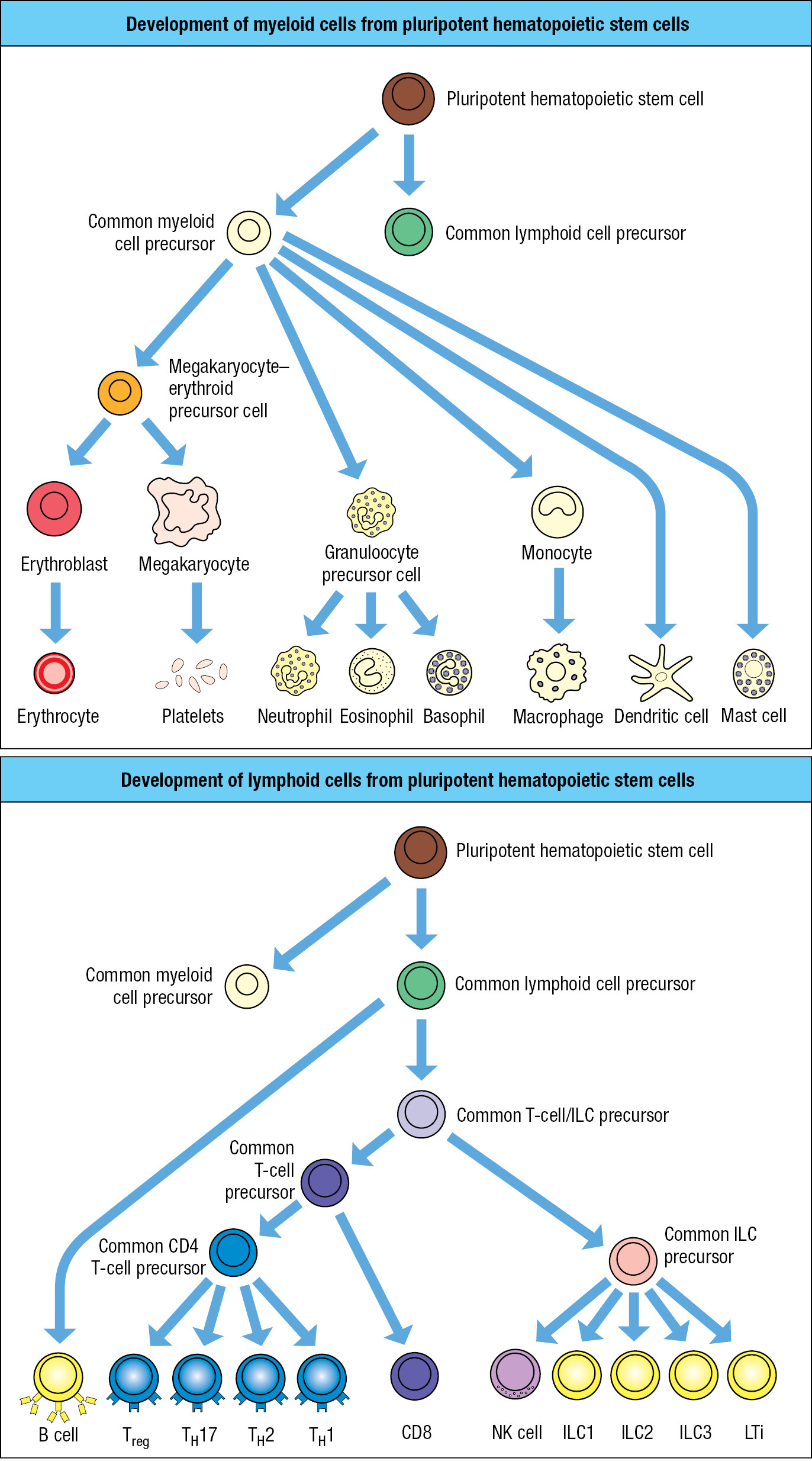
Cell type | Percentage of total leukocytes (%) |
---|---|
Neutrophil Eosinophil Basophil Monocyte Lymphocyte |
40–75 1–6 <1 2–10 20–50 |
The myeloid precursor cell (also called the myeloid progenitor) gives rise to the myeloid cell lineage. One group of myeloid cells is the granulocytes, which comprise neutrophils, eosinophils, and basophils. These effector cells of innate immunity have prominent cytoplasmic granules filled with reactive biochemicals that kill microorganisms and enhance inflammation. The granulocytes are also known as polymorphonuclear leukocytes because of their irregularly shaped nuclei with two to five lobes. The most abundant white blood cell by far is the neutrophil (Figure 1.14), which specializes in the capture and killing of microorganisms; the process is called phagocytosis. Such cells are called phagocytes, with neutrophils being the most lethal. They rapidly mobilize to enter infected tissue and can function under the anaerobic conditions that prevail in damaged tissue. Neutrophils are short-lived and die within the infected tissue, forming pus, the creamy stuff of pimples and boils (Figure 1.15). The eosinophil, a less abundant granulocyte than the neutrophil, specializes in defending the body against intestinal parasites, particularly helminth worms. The basophil, the rarest of the granulocytes, initiates the anti-parasite response and acts more like a regulatory cell than an effector cell. The names of the granulocytes refer to the reactivity of their cytoplasmic granules with histological stains. The eosinophil’s granules contain basic substances that bind the acidic stain eosin, the basophil’s granules contain acidic substances that bind the basic stain hematoxylin, and the neutrophil’s granules bind neither acidic nor basic stains.
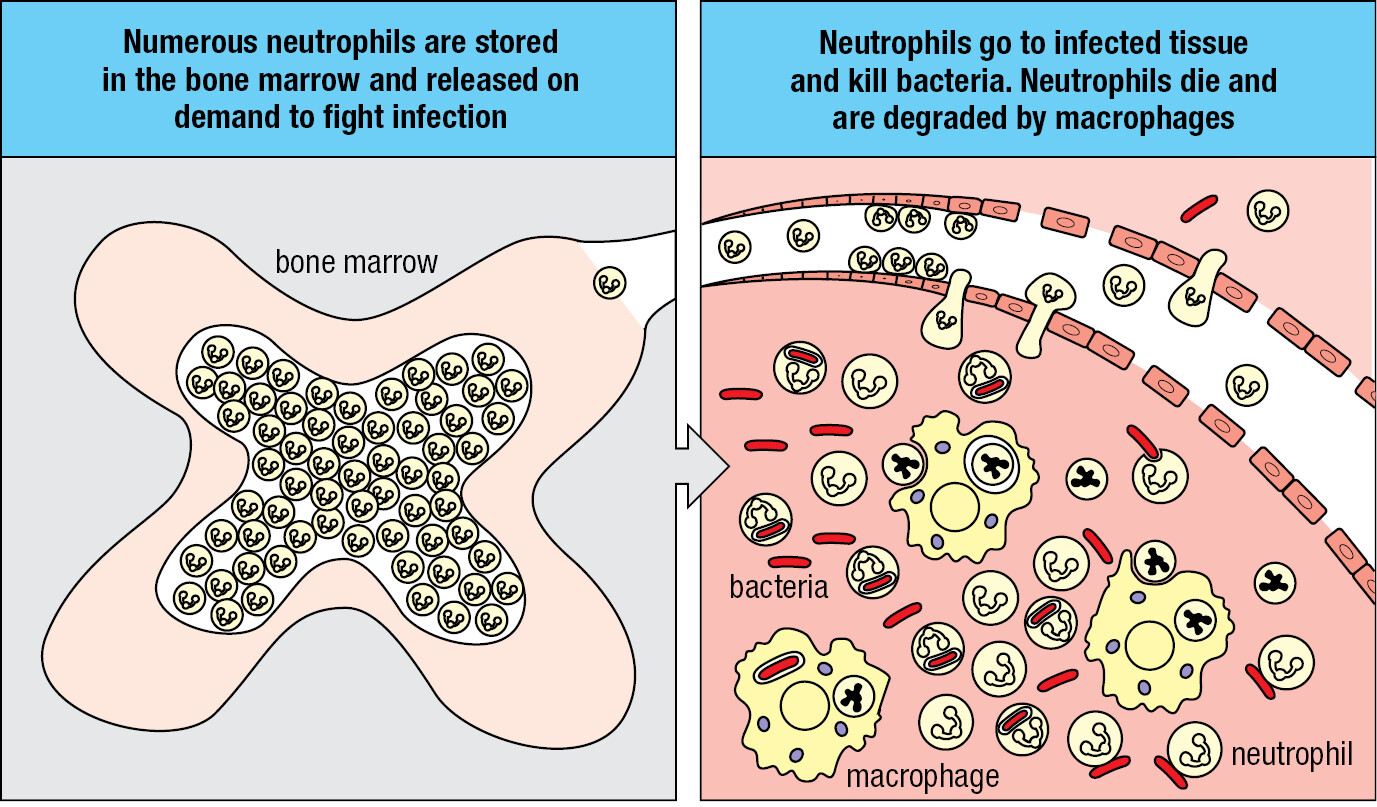
A second group of myeloid cells consists of the macrophages, monocytes, and dendritic cells. Tissue macrophages are large, irregularly shaped phagocytic cells having an extensive cytoplasm with numerous vacuoles containing engulfed material in the process of being broken down and digested (Figure 1.16). During early development in utero, tissue macrophages derived from embryonic stem cells move to a tissue, where they become the tissue-resident macrophages. Infection of a tissue is first detected by the resident macrophages, which are activated to kill pathogens and recruit neutrophils to the innate immune response. Numerous neutrophils die in the infected tissue, where the macrophages dispose of them. The name macrophage means ‘large phagocyte,’ and the neutrophil, historically called the microphage, is the ‘small phagocyte.’ Neutrophils are the short-lived infantry of innate immunity, whereas macrophages are the long-lived commanders who orchestrate the local response to infection. As part of their response to the pathogen, macrophages secrete the inflammatory cytokines that recruit neutrophils and other leukocytes into the infected area.
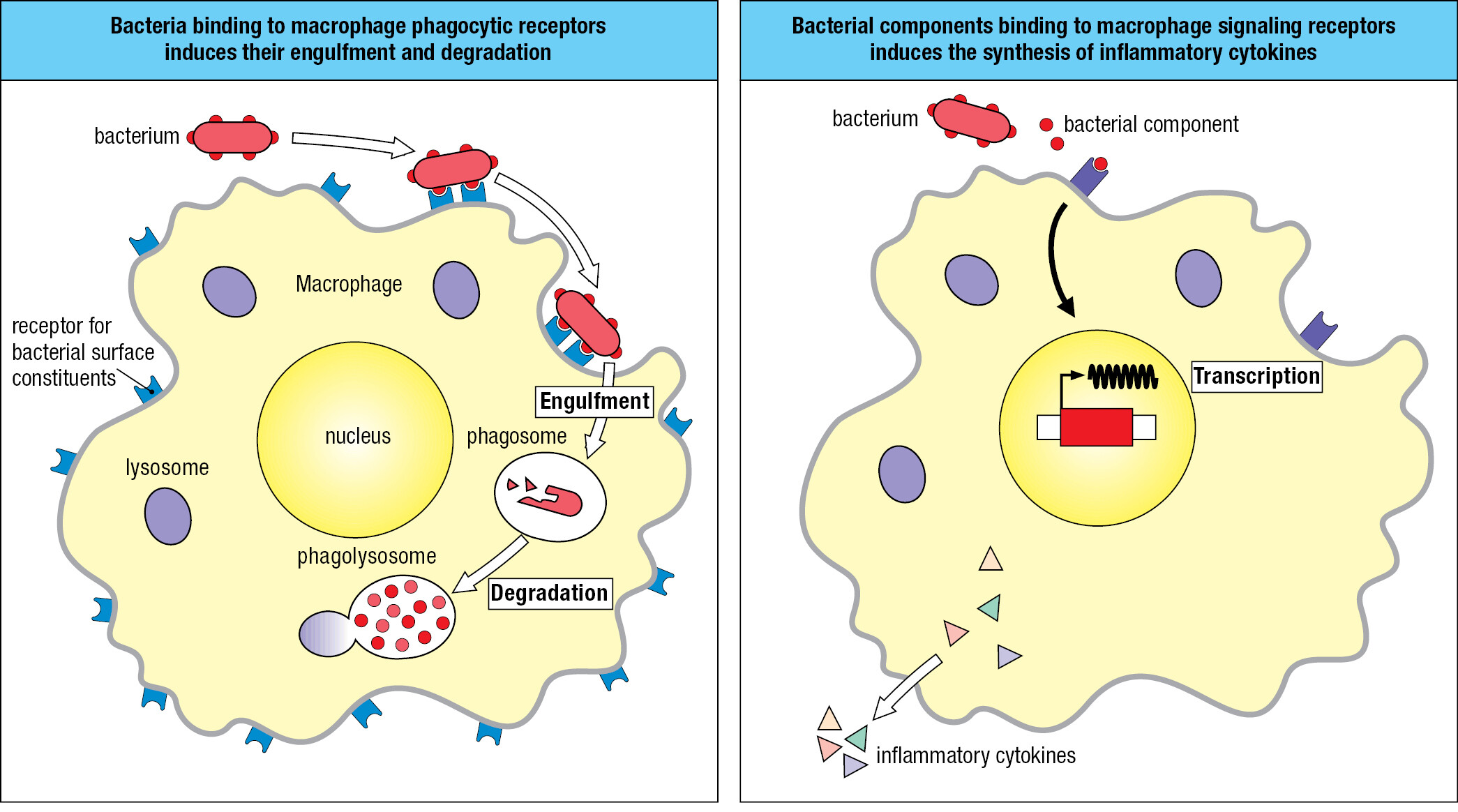
Monocytes are leukocytes that circulate in the blood. They are distinguished from granulocytes by their larger size, distinctive indented nucleus, and uniform appearance: hence the name monocyte. Macrophages recruit monocytes to infected tissue, where the monocytes differentiate into macrophages and reinforce the resident macrophages. Although the resident macrophages of healthy tissue are self renewing, in the presence of infection and extensive tissue damage they can be overwhelmed by the sheer amount of dead pathogens and neutrophils that need to be phagocytosed; at such a point the macrophages too can die. Once the pathogen has been vanquished and is no longer a threat, the tissue will be repaired and repopulated with monocytes, which differentiate in the tissue to become tissue-resident macrophages.
With a distinctive star-shaped morphology, dendritic cells are residents of the body’s tissues and have properties and functions in common with macrophages. The critical function of the dendritic cell is one of decision: to determine whether, and when, the innate immune response needs reinforcement with an adaptive immune response. When adaptive immunity is required, dendritic cells within the infected tissue gather up a cargo of intact and degraded pathogens and take them to lymphoid tissues, where they initiate the adaptive immune response to the pathogen.
The mast cell is resident in all connective tissues. It has granules like those of the basophil but is otherwise not a close relative. The blood-borne progenitor of the mast cell has yet to be discovered and remains a mystery. The mast-cell granules contain chemical mediators that cause violent spasms of smooth muscle that forcefully eject parasites from the respiratory tract and the gut.
The lymphoid precursor cell (also called the lymphoid progenitor) gives rise to the lymphoid lineage of leukocytes. Morphologically, two populations of blood lymphocytes are distinguished: large lymphocytes with a granular cytoplasm, and small lymphocytes with little cytoplasm. The large granular lymphocytes include the natural killer cells (NK cells), which are cytotoxic lymphocytes of innate immunity that respond to viral infections. Macrophages recruit NK cells to infected tissues, where they stop the spread of infection by killing virus-infected cells and secreting cytokines that impede viral replication. Other large lymphocytes of innate immunity are the innate lymphoid cells ILC1, 2, and 3 and LTi (see Figure 1.13). They are resident in tissues and do not circulate between blood and lymph. ILCs secrete cytokines that help to activate the responses of myeloid cells to infection.
The quiescent small lymphocytes mediate all adaptive immune responses. They circulate in an immature and functionally inactive form, hence their small size. Recognition of a pathogen by pathogen-specific receptors on small lymphocytes (see Section 1-5) drives a process of cellular selection, growth, and differentiation that in 1–2 weeks produces a powerful and tailored response aimed at an invading organism.
1-7Immunoglobulins and T-cell receptors are the antigen receptors of adaptive immunity
Although the small lymphocytes are morphologically indistinguishable, they comprise many sublineages that are distinguished by their cell-surface glycoproteins and immunological functions. The fundamental distinction is between B lymphocytes and T lymphocytes, usually called B cells and T cells. The cell-surface pathogen receptors of B cells are called B-cell receptors and are immunoglobulins; those of T cells are called T-cell receptors and are different from, but structurally related to, immunoglobulins. Plasma cells are effector B cells. They secrete a soluble form of the B-cell receptor—the antibody (Figure 1.17). T-cell receptors are only expressed as cell-surface receptors and not as soluble forms.
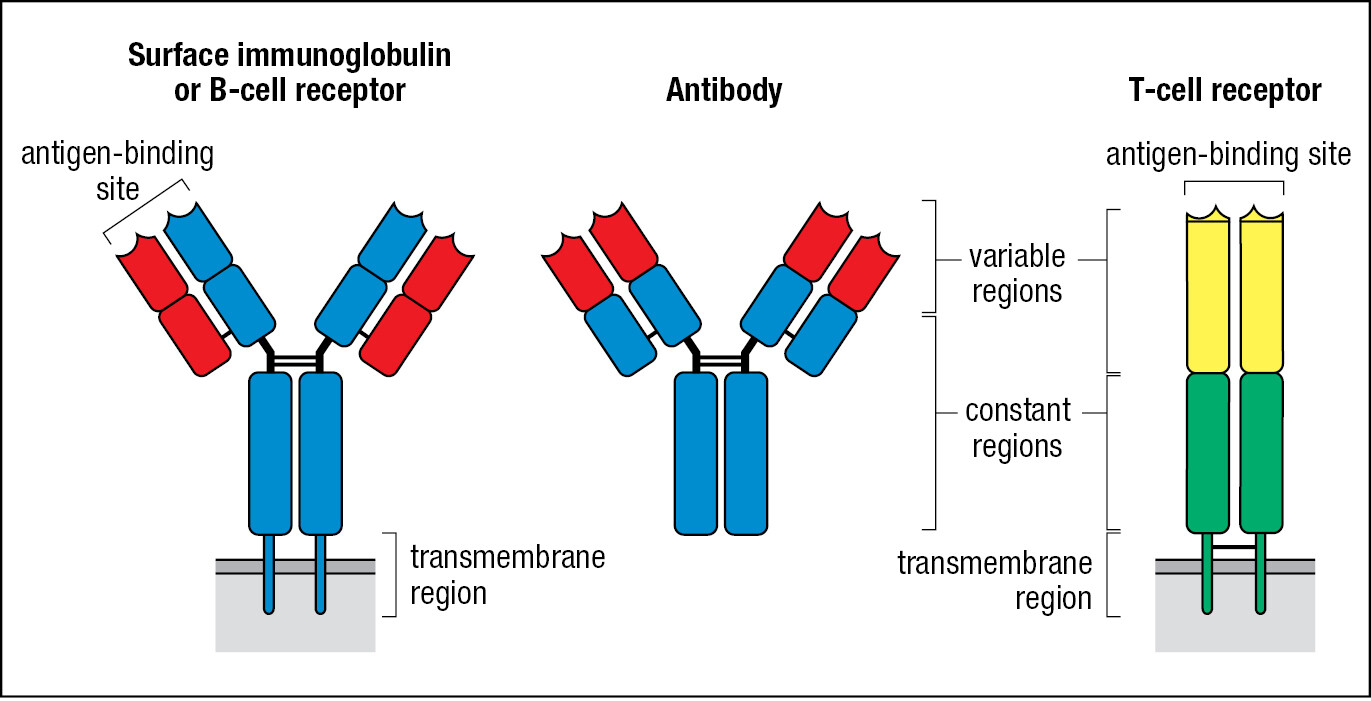
Immunoglobulins are composed of two identical heavy chains and two identical light chains. T-cell receptors are composed of just two protein chains, an α chain and a β chain. The genes encoding the immunoglobulin heavy and light chains and the T-cell receptor α and β chains are exceptional—qualitatively different from all other human genes. The germline does not contain functional immunoglobulin and T-cell receptor genes, but instead there are arrays of alternative gene segments that must be cut and spliced to produce the functional gene, a process called gene rearrangement. The enzymes involved are error-prone and introduce somatic mutations that further increase the sequence diversity of the immunoglobulin and T-cell receptor chains. Because of the unusual properties of these genes, each B cell expresses only one form of heavy chain and one form of light chain, but within a person’s population of B cells there are billions of different heavy and light combinations. Likewise, each T cell expresses one α and one β chain, but in a person’s T-cell population there are billions of different α- and β-chain combinations. The extent of this diversity enables a specific adaptive immune response to be made against any pathogen, including one such as SARS-CoV-2 that is new to the human species. The structures of these receptors and how they are generated during lymphocyte development are discussed in Chapters 4–7.
Any macromolecule, virus particle, or cell-surface component that is recognized and bound by an immunoglobulin or T-cell receptor is referred to as its antigen. Cell-surface immunoglobulins and T-cell receptors are also referred to as the antigen receptors of their respective lymphocytes. Differences in the amino acid sequences of the variable regions of immunoglobulins and T-cell receptors create a vast variety of binding sites that are specific for different antigens and pathogens. Because each B cell and T cell expresses just one form of antigen receptor, the adaptive immune response made against one pathogen provides no immunity to an unrelated pathogen. Thus antibodies made after measles vaccination bind to measles virus but not to influenza virus, whereas antibodies made after influenza vaccination bind to influenza virus but not to measles virus.
1-8On binding specific antigen, B cells and T cells divide and differentiate into effector cells
When a B cell encounters the specific antigen recognized by its B-cell receptor, the B cell is activated to divide and differentiate into antibody-producing plasma cells. The synthesis and secretion of antibody is the principal effector function of B cells (discussed in Chapter 9). By contrast, antigen-activated effector T cells are of different functional types that are distinguished by their surface glycoproteins, the cytokines they respond to, the cytokines they secrete, and the type of target cell they interact with (discussed in Chapter 8). The main subdivision of effector T cells is defined by the cell-surface expression of either the CD4 or CD8 co-receptor protein (the CD designation is an acronym for ‘cluster of differentiation’ and is a means of unambiguously naming the numerous cell-surface proteins that have historically been given many different names). At the T-cell surface, the co-receptor associates with the T-cell receptor and signaling components to form a complex that recognizes and responds to specific antigen. T cells expressing the CD8 co-receptor are cytotoxic T cells that kill cells infected with a virus or bacterium. These cytotoxic T cells of adaptive immunity have similar effector functions to the NK cells of innate immunity (Figure 1.18). T cells expressing the CD4 co-receptor are known as helper T cells because they secrete cytokines that enable other cells to become fully activated effector cells.
Effector lymphocytes of innate and adaptive immunity | |||
---|---|---|---|
Innate immunity | |||
NK cell | ILC1 | ILC2 | ILC3 |
Type 1 immunity | Type 1 immunity | Type 2 immunity | Type 3 immunity |
Cell-mediated cytotoxicity Intracellular infections Viruses and some bacteria | Inflammatory macrophage activation Extracellular infections Bacteria | Noninflammatory macrophage activation Intestinal parasite infections | Promotion of phagocytosis and secretion of antimicrobial peptides |
IFN-γ | IFN-γ | IL-4, IL-5, IL-13 | IL-22, IL-17 |
CD8 T cell | TH1 CD4 cell | TH2 CD4 cell | TH17 CD4 cell |
Adaptive immunity |
The TH1 subset of helper T cells activates macrophages to increase phagocytosis and to kill those phagocytosed pathogens that resist the usual processes of intracellular degradation. TH17 cells activate neutrophils to phagocytose and eliminate extracellular bacteria. The TH2 subset responds to parasite infections by secreting cytokines that activate mast cells, basophils, and eosinophils. The mediators released by these cells induce contractions of smooth muscle that eject parasitic worms and protozoans from the gut and respiratory tracts. These same mechanisms cause the allergic reactions to pollen, nickel, and other harmless substances. Cytokines secreted by TFH cells induce the differentiation of antigen-activated B cells to become antibody-secreting plasma cells. Once an infection is terminated, another subset of CD4 T cells, Treg cells, closes down the effector CD4 and CD8 T-cell responses to prevent further inflammation and tissue damage.
In the past 10 years, subpopulations of innate lymphoid cells have been shown to contribute to innate immunity in ways that parallel the TH1, TH2, and TH17 subsets of helper CD4 T cells. The helper functions of ILCs complement the cytotoxic activity of NK cells. The ILCs reside in tissues, where most infections occur, and do not circulate in the blood like NK cells (see Figure 1.18).
1-9B cells and T cells recognize different categories of microbial antigens
The antigen-binding sites of B-cell receptors and antibodies recognize carbohydrates, glycoproteins, proteoglycans, and glycolipids on the surface of microbial pathogens. In this manner, B-cell receptors and antibodies recognize the native conformations and three-dimensional structures of microbial macromolecules. The part of the outer surface of an antigenic macromolecule that interacts directly with an antibody or B-cell receptor is called the epitope.
T-cell receptors recognize microbial products in a very different way than B-cell receptors and antibodies. The epitopes recognized by T-cell receptors are short peptides of 8–16 amino acids and are produced in phagocytic myeloid cells by the degradation of pathogenic bacteria and in any human cell that is infected with a virus and making viral proteins. T-cell receptors cannot recognize a peptide antigen alone, but require it to be bound by a human glycoprotein called a major histocompatibility complex molecule, or MHC molecule. In this context, the MHC molecule is said to ‘present’ the peptide antigen to the T-cell receptor. There are a number of different types of MHC molecule, and the genes that encode them are the most polymorphic of human genes; that is, each gene exists in many different variants within the human population. The particular set of MHC molecules a person expresses is known as that person’s tissue type, and because of the extensive genetic polymorphism of the MHC genes, the tissue type differs in several respects between individuals. Immune responses against differences in tissue type are the principal cause of rejection in the clinical transplantation of organs and tissues.
Two classes of MHC molecule provide defense against different types of infection (Figure 1.19). MHC class I defends against intracellular infections; that is, all viral and some bacterial infections. MHC class II defends against extracellular infections. Because all human cells are susceptible to viral infection, MHC class I molecules are expressed by all cells. On the other hand, the expression of MHC class II molecules is not needed by most cells, and their expression is restricted to professional antigen-presenting cells: the macrophages, dendritic cells, and B cells. In the interactions between effector T cells and their target cells, CD8 binds to MHC class I but not to MHC class II. Conversely, CD4 binds to MHC class II but not to MHC class I. Consequently, all CD8 cells are cytotoxic T cells, and all CD4 T cells are helper T cells.
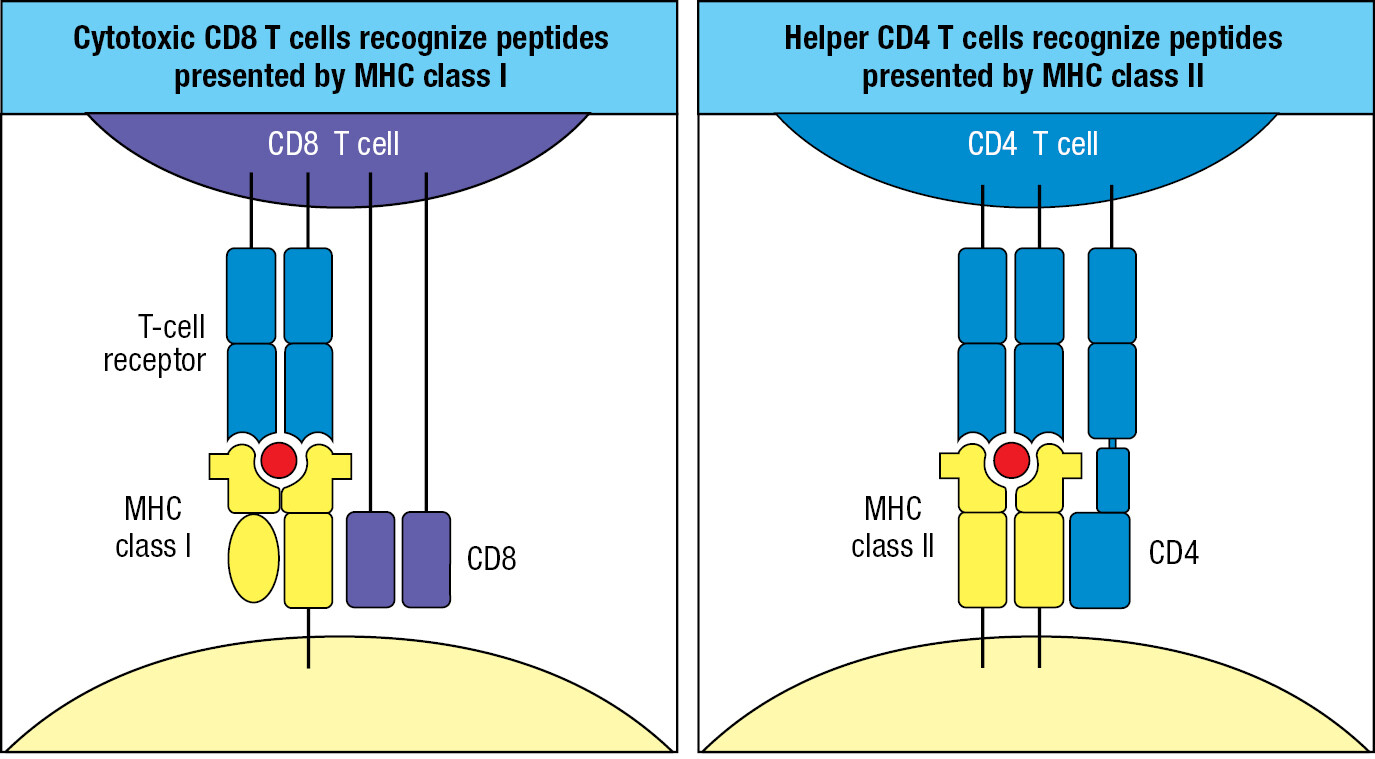
1-10Antibodies binding to a pathogen cause its inactivation or elimination
The antibodies secreted by plasma cells circulate in the blood, enter infected tissues, and bind to the surface antigens of pathogens. Such bound antibody can limit infection by interfering with pathogen growth and replication and with pathogen interactions with human cells. Such impedance of a pathogen by an antibody is called neutralization. An essential property of any influenza vaccine is to stimulate the formation of neutralizing antibodies that will coat the virus and prevent it from infecting human cells. With a different strategy, the lethal effects of bacterial toxins can be abrogated with antibody that binds the toxin and covers up its active site (Figure 1.20). The immunity conferred by antibodies is called humoral immunity. Humors are the body fluids, and for many years they were the only source of antibodies for use in medicine and research.
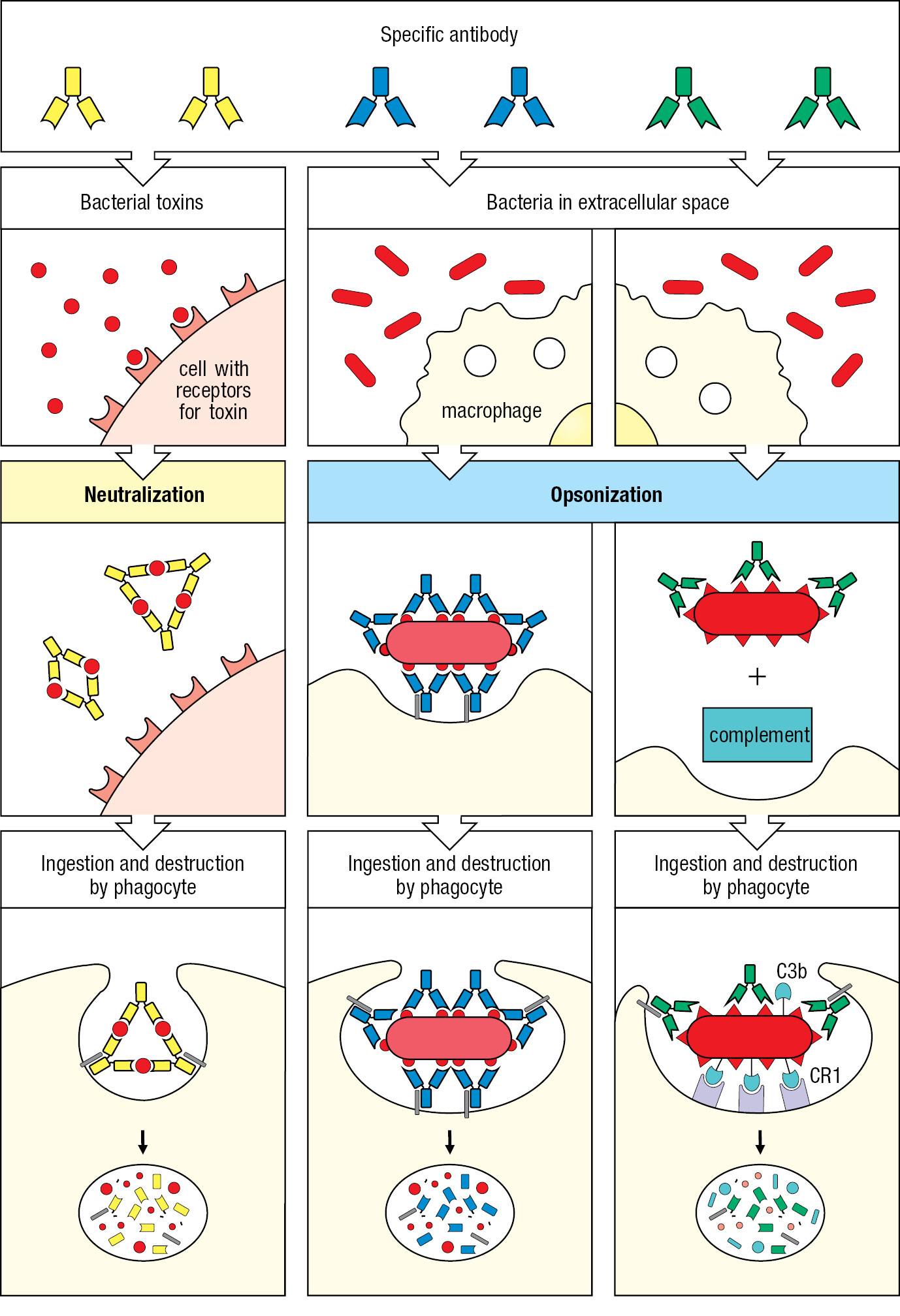
Antibodies can facilitate the engulfment and destruction of extracellular microorganisms and toxins by phagocytes. Because pathogen surfaces consist of a relatively few types of macromolecule, the antigenic macromolecules are present at high density. An antibody specific for one of these antigens can be sufficient to coat the entire pathogen surface. Phagocytes, such as neutrophils and macrophages, have cell-surface receptors that bind the antibody at a site away from its antigen-binding site, which is attached to the pathogen. When numerous receptors on the phagocyte engage an equal number of antibodies bound to the pathogen’s antigens, the pathogen becomes subject to rapid engulfment and death by digestion. Bacteria coated with antibody are far more efficiently phagocytosed than uncoated bacteria. The coating by antibody, leading to enhancement of phagocytosis, is called opsonization (see Figure 1.20).
1-11Most lymphocytes are present in specialized lymphoid tissues
The lymphocytes studied by immunologists have usually been obtained from the peripheral blood of patients and blood donors. However, only a small fraction of lymphocytes are ever in the blood, which they use to travel between tissues. The great majority of lymphocytes are present in specialized lymphoid tissues. The main lymphoid tissues are bone marrow, thymus, spleen, adenoids, tonsils, appendix, lymph nodes, and Peyer’s patches (Figure 1.21). Less-organized lymphoid tissue is also found lining the extensive mucosal surfaces of the respiratory, gastrointestinal, and urogenital tracts. The lymphoid tissues are divided into two functional types. Primary lymphoid tissues (sometimes called central lymphoid tissues) are where lymphocytes develop and mature to the stage at which they are able to recognize and respond to a pathogen. The bone marrow and the thymus are the only primary lymphoid tissues in humans. B and T lymphocytes both originate from lymphoid precursor cells in the bone marrow (see Figure 1.13). B cells complete their maturation in the bone marrow before entering the circulation. By contrast, T cells leave the bone marrow at an early stage in their development and migrate in the blood to the thymus, where they mature. All other lymphoid tissues are secondary lymphoid tissues (sometimes called peripheral lymphoid tissues); these are the sites where mature lymphocytes encounter pathogen-derived antigens and are activated to become effector cells that attack and eliminate the microbial invader.
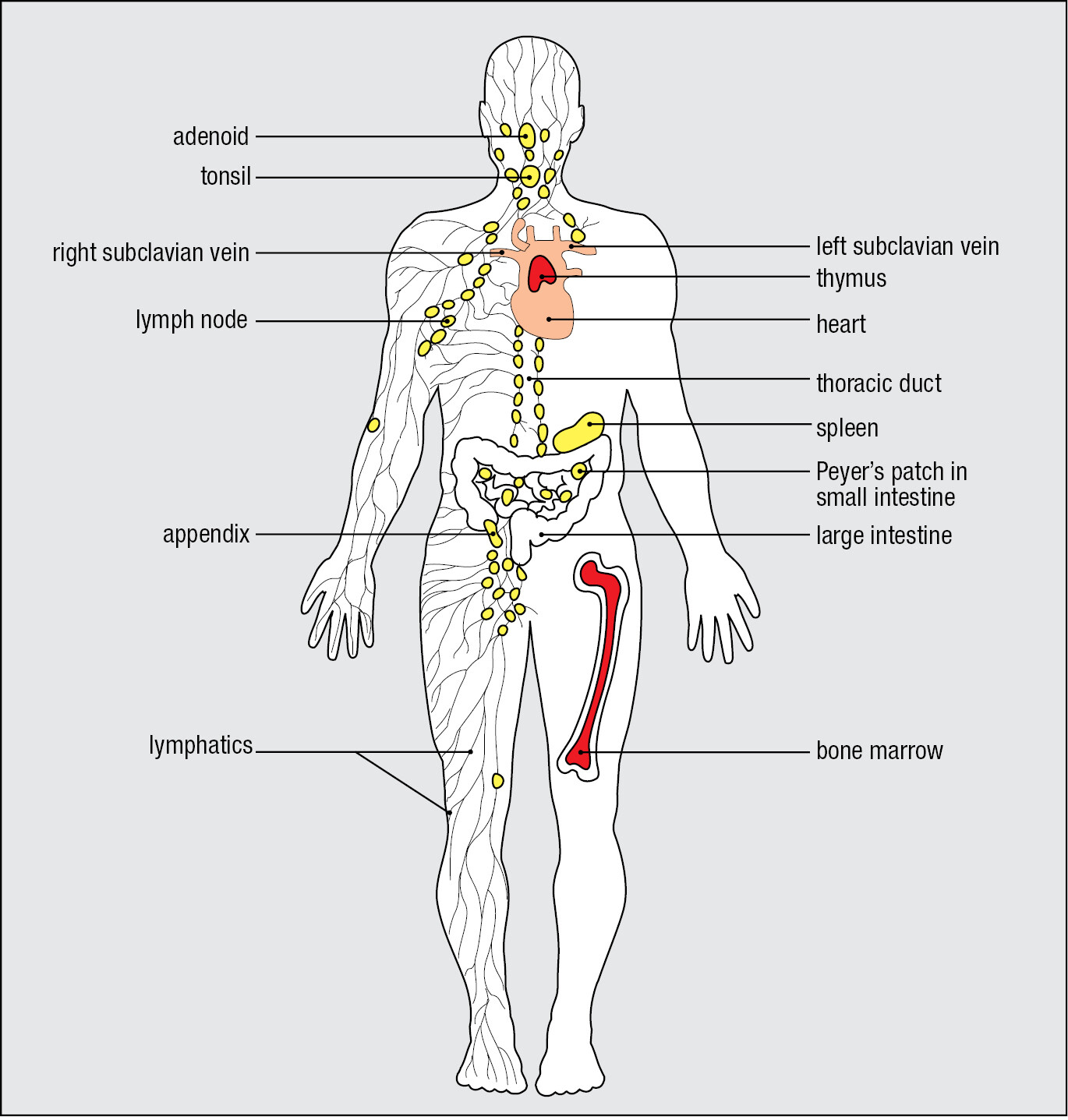
Lymph nodes are placed at the junctions of an extensive network of lymphatic vessels called the lymphatics, which originate in connective tissues throughout the body and collect the plasma that leaks out of blood vessels to become the extracellular fluid. The lymphatics eventually return this fluid—the lymph—to the blood. The main route for this return is through the thoracic duct, which empties into the left subclavian vein in the neck. Unlike blood, the lymph is not pumped, so its flow is sluggish. One-way valves within lymphatic vessels, and lymph nodes at their junctions, ensure that the net movement of the lymph is always in a direction away from the peripheral tissues and toward the ducts in the upper body where the lymph empties into the blood. The flow of lymph is driven by the constant movements of one part of the body against another. In the absence of these movements, for example, when a person is confined to bed, the flow of lymph slows and fluid accumulates in tissues, causing the swelling known as edema.
B cells and T cells are distinguished from all other blood cells by the way they move around the body using both blood and lymph. Lymphocytes are the only cells present in lymph in any numbers; hence their name. After small lymphocytes have developed in the primary lymphoid tissues, they then enter the bloodstream. On reaching the blood capillaries that invest a lymph node (or other secondary lymphoid tissue), small lymphocytes are able to leave the blood and enter the tissue of the lymph node. If a lymphocyte becomes activated by a pathogen it remains in the lymph node; otherwise it spends some time there, but then leaves in the efferent lymph—the lymph leaving a lymph node—and eventually returns to the blood. With this lifestyle, the population of lymphocytes within a lymph node is in a continual state of flux, with new arrivals from the blood forcing older tenants into the efferent lymph. This pattern of movement between blood and lymph is called lymphocyte recirculation (Figure 1.22). It allows the lymphocyte population to monitor the secondary lymphoid tissues for any evidence of infection. An exception to this pattern is the spleen, which has no lymphatics. Lymphocytes both enter and leave the spleen in the blood.
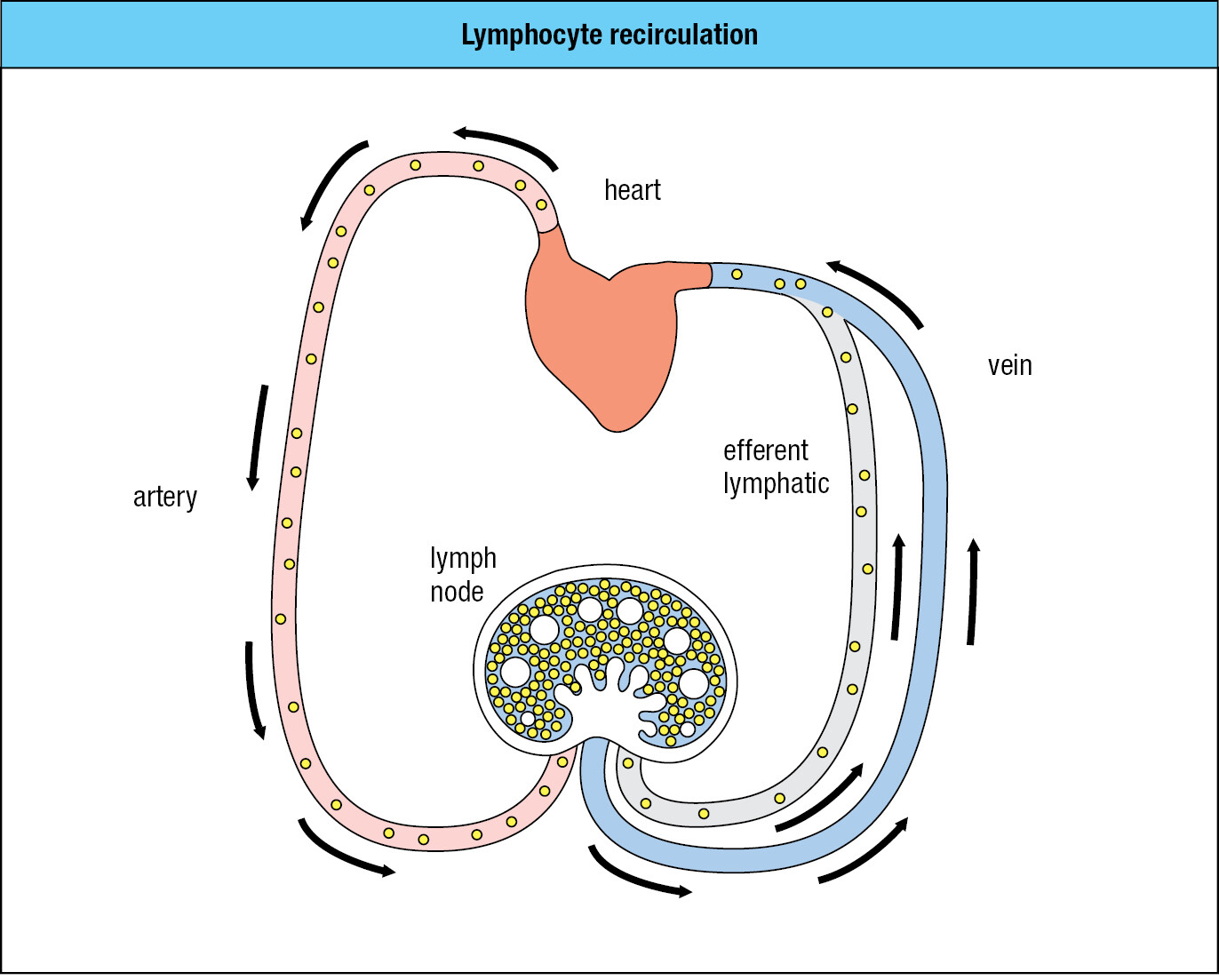
The secondary lymphoid tissues are dynamic: lymphocytes constantly arrive from the blood and depart in the lymph. At any one time, only a minute fraction of lymphocytes are in the blood and lymph; the large majority of them are in lymphoid tissues.
1-12Adaptive immunity is initiated in secondary lymphoid tissues
Experimental infection of volunteers with cold viruses showed that a large first dose of virus is necessary to create an infection and cause disease. To establish infection, a microorganism must colonize a tissue to the extent that it overwhelms the local innate immune response. Common sites of infection are the connective tissues that pathogens penetrate as a result of skin wounds. From such sites, dendritic cells that are either pathogen-infected or loaded with the pathogen’s antigens are carried in the lymph through the lymphatics to the nearest lymph node. This lymph node is the draining lymph node, which collects all particulate and potentially dangerous materials and prevents them from entering the circulation (Figure 1.23).
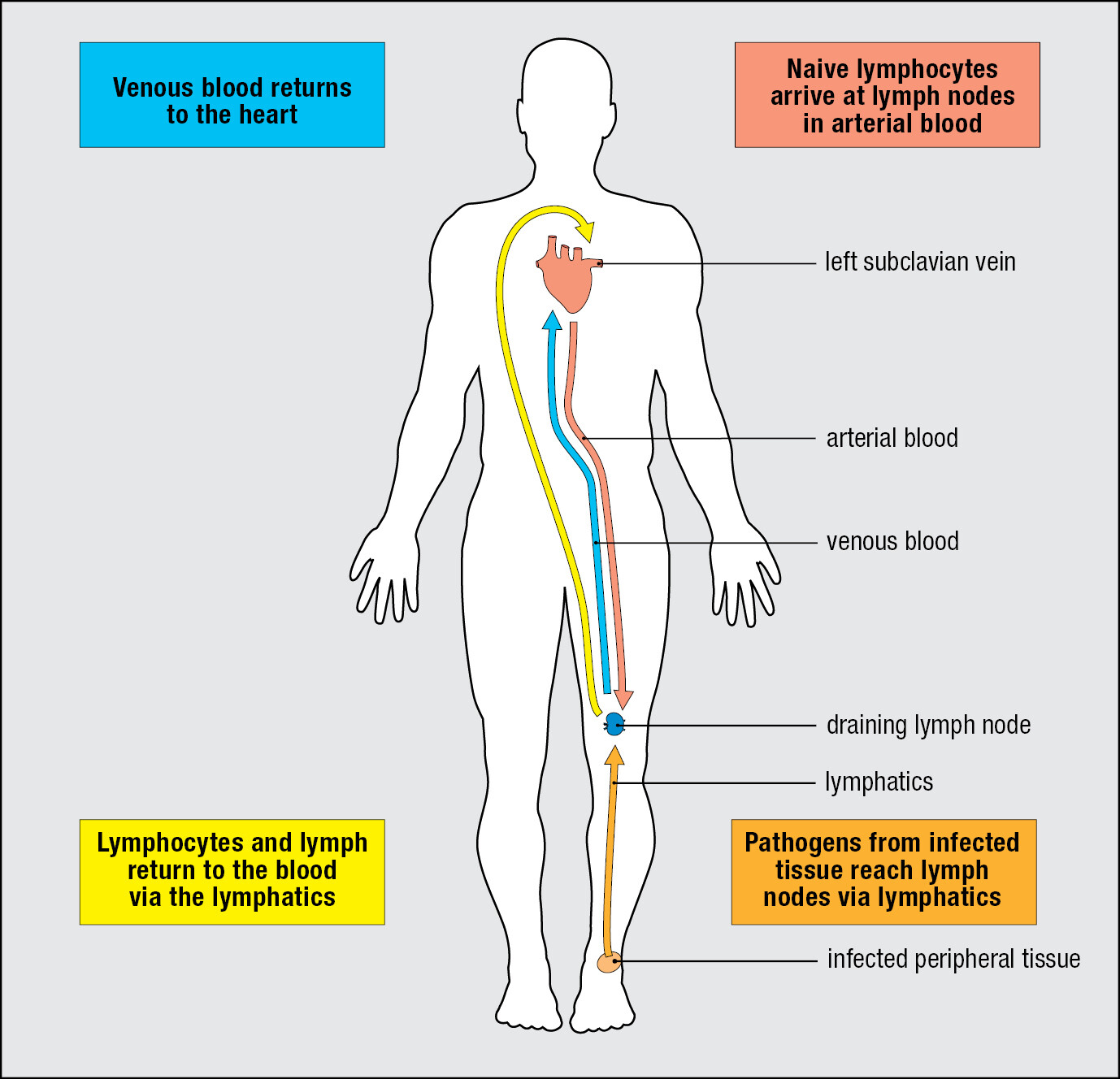
The anatomy of the lymph node provides a meeting place where lymphocytes coming from the blood encounter pathogens and antigens brought from the infected connective tissue (Figure 1.24). Arriving lymphocytes are segregated, T cells to T-cell areas and B cells to lymphoid follicles. Pathogens and antigen-laden dendritic cells enter the lymph node in an afferent lymphatic vessel. Several of these converge at the node, and a single efferent lymphatic vessel carries lymph out of the node. As the lymph passes through the node, dendritic cells stay in the node, allowing pathogens and other extraneous materials to be extracted by the resident macrophages. This prevents infectious organisms from reaching the blood and provides the lymph node with pathogen-derived antigens and antigen-bearing dendritic cells.
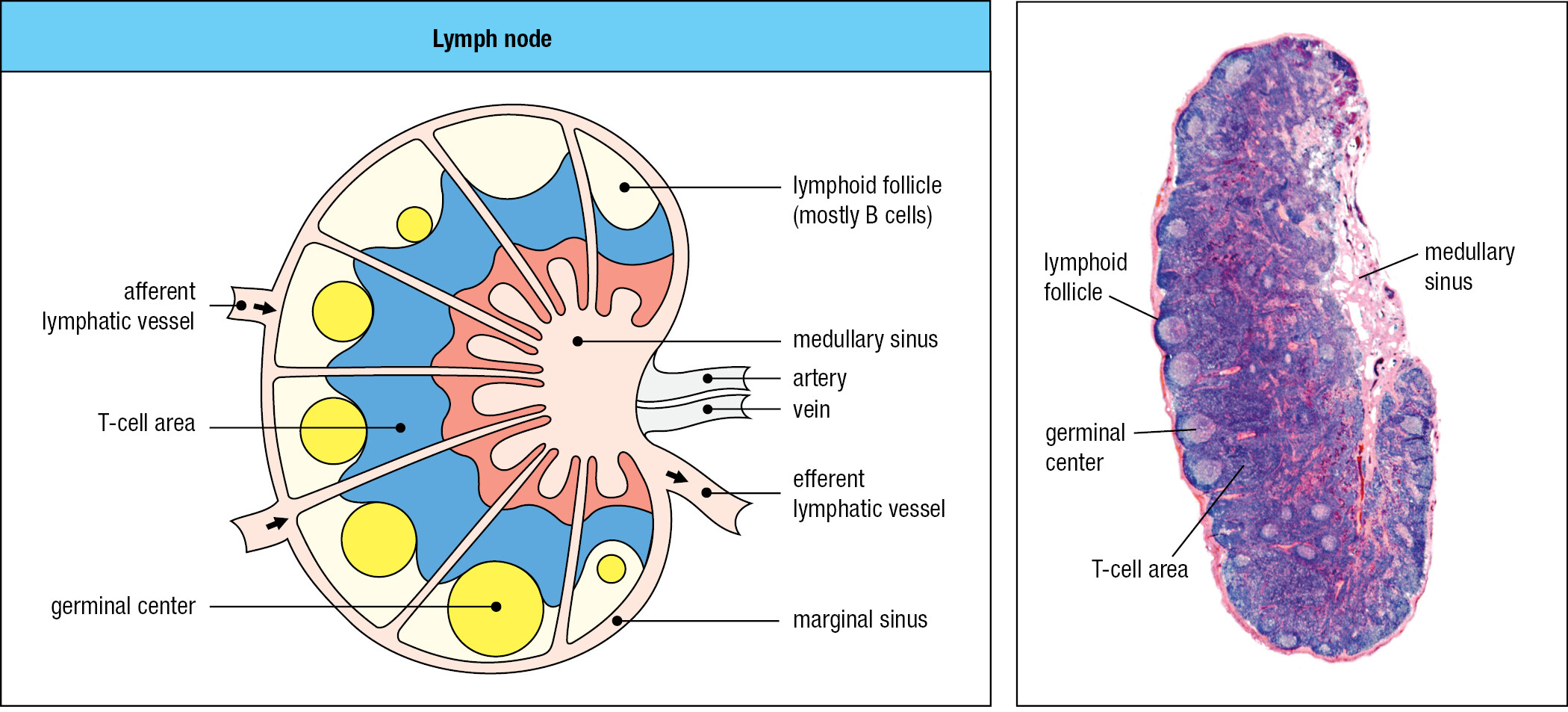
In the lymph node, the small fraction of B and T cells that have receptors specific for the pathogen’s antigens are stimulated to divide and differentiate into effector cells. Activated pathogen-specific B cells proliferate in each follicle, forming a dense spherical structure, the germinal center. The pathogen-specific T cells are activated by antigen-bearing dendritic cells, some of which then move to the associated lymphoid follicle, where they help antigen-activated B cells to become plasma cells secreting antibody. As a consequence of the lymphocyte activation, proliferation, and differentiation, the lymph node swells in size and can be painful. Other effector T cells and the antibodies secreted by plasma cells are carried in the efferent lymph and the blood to the infected tissue (Figure 1.25). There, the effector cells and antibodies of adaptive immunity synergize with the effector cells and proteins of innate immunity to subdue the infection. Recovery from infection involves the clearance of infectious organisms from the body and repair of the damage caused by the pathogen and the immune response. Cure is not always possible. Infection can overwhelm the immune system, with death as the consequence. In the United States, some 36,000 deaths each year are associated with influenza virus infections. For other pathogens, the infection persists, but its pathological effects are controlled by the adaptive immune response, as usually occurs with herpesvirus infections.
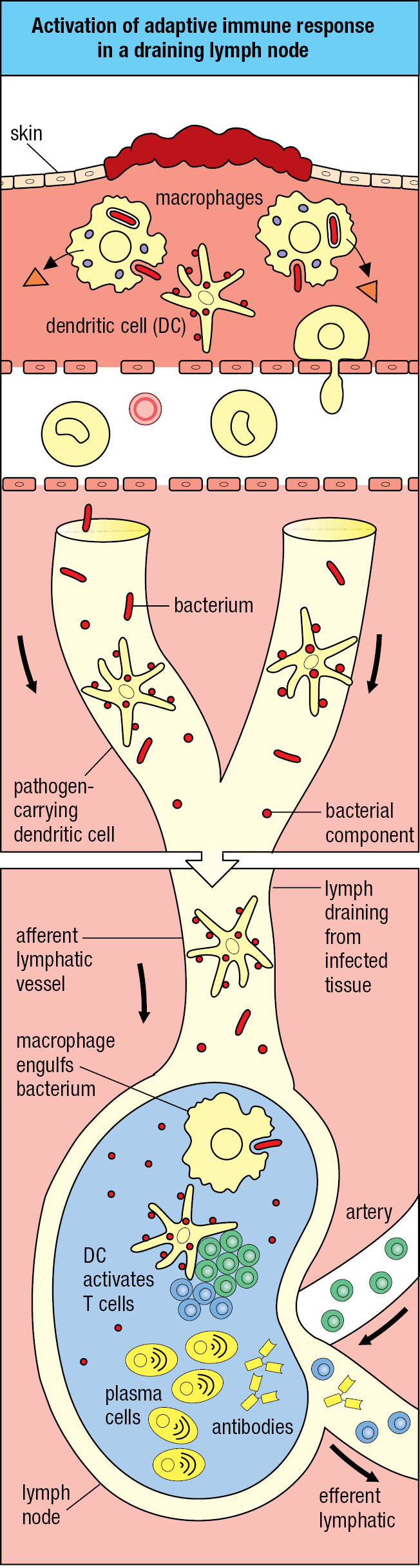
1-13The spleen provides adaptive immunity to blood infections
Pathogens can enter the blood directly, as occurs when blood-feeding insects transmit disease or when the lymph nodes draining infected tissue fail to remove all microbes before returning the lymph to the blood. The spleen is the organ that filters the blood to remove damaged and senescent erythrocytes. The spleen’s other function is that of a secondary lymphoid tissue that defends the body against blood-borne pathogens. Any microorganism that gets into the blood is a potential pathogen and source of systemic infection. Microorganisms and microbial products in the blood are taken up by splenic macrophages and dendritic cells, which then stimulate the B and T cells that arrive in the spleen from the blood. The spleen comprises two types of tissue: the red pulp, where red blood cells are monitored and elderly or damaged ones removed, and the white pulp, a secondary lymphoid tissue. The organization and functions of white pulp are like those of a lymph node, the main difference being that the spleen has no lymphatics. Pathogens and lymphocytes both enter and leave the spleen in the blood (Figure 1.26).
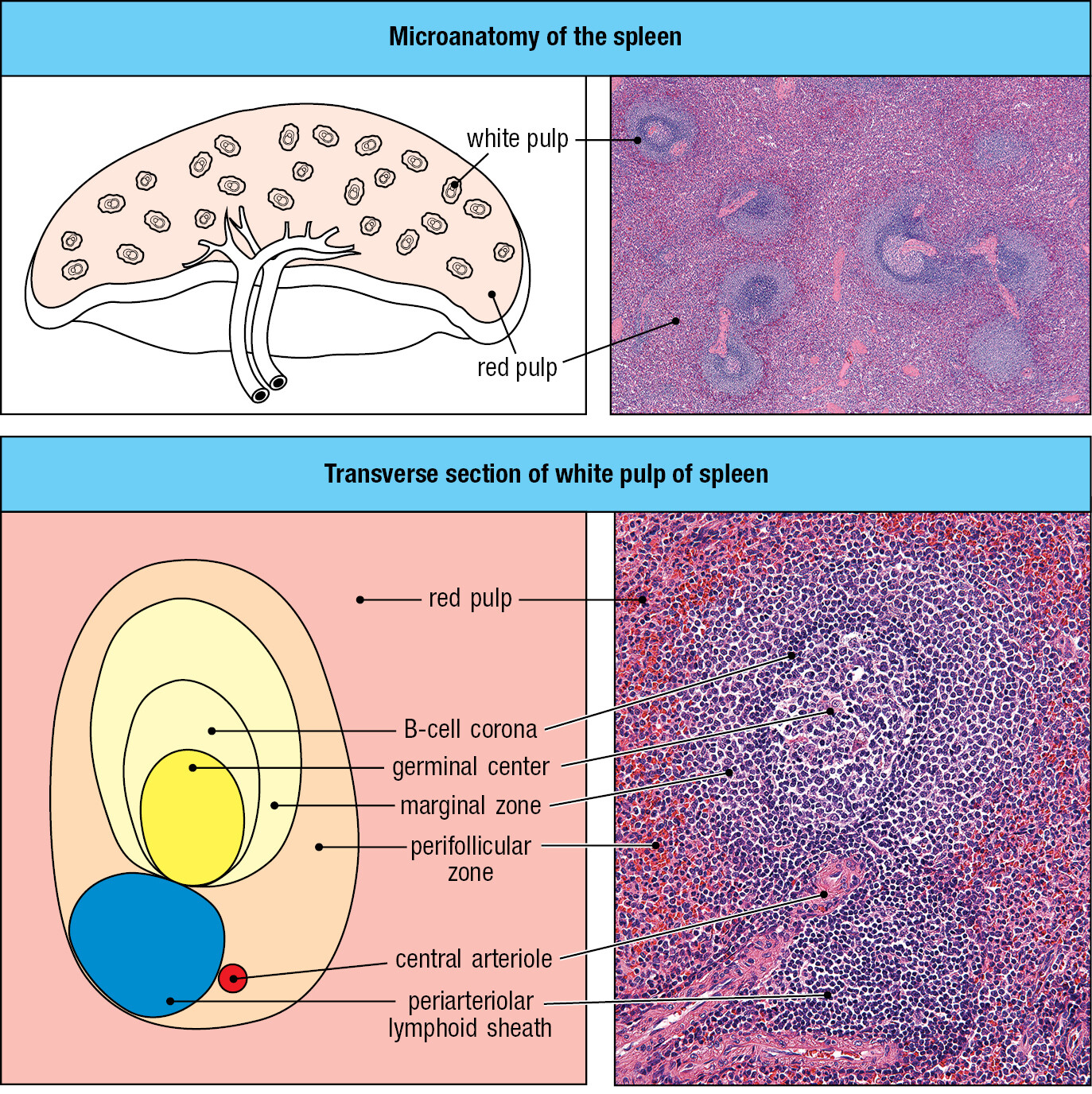
Some children are born without a spleen, the condition called asplenia (Figure 1.27). It is caused by mutation of the gene encoding ribosomal protein SA. The mutant allele is dominant, so inheritance of one mutant allele produces asplenia. All other organs appear normal in individuals with the condition. Children with asplenia are highly susceptible to infections, particularly with bacteria such as Streptococcus pneumoniae (see Figure 1.3d) or Haemophilus influenzae that have thick polysaccharide capsules. Children with asplenia can be protected against these life-threatening infections if their condition is diagnosed early in life, and they are vaccinated against S. pneumoniae and H. influenzae. For best effect, the vaccines are injected subcutaneously, which stimulates an immune response in the draining lymph nodes, which are normal in asplenic individuals. Asplenia is an immunodeficiency disease, a condition in which the inheritance of a mutant gene leads to a defect in immunological function (immunodeficiency is discussed in Chapter 13). The discovery and study of immunodeficiency diseases has largely been the work of pediatricians, because these conditions usually appear in early childhood. Unlike asplenia, most immunodeficiencies are recessive and become manifest only in children with two mutant alleles of the relevant gene.
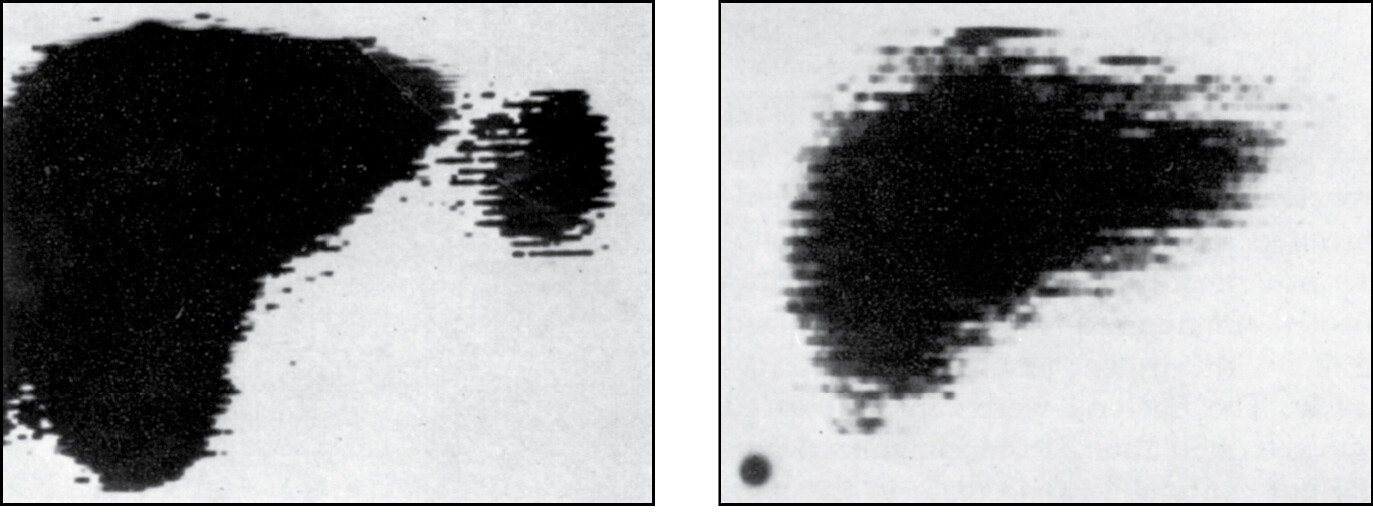
1-14Most of the body’s secondary lymphoid tissue is associated with the gut
The sites in the body that contain the largest and most diverse populations of microorganisms are the respiratory and gastrointestinal tracts. The extensive mucosal surface of these tissues makes them vulnerable to infection. For their defense these mucosal tissues are heavily invested with secondary lymphoid tissue, known collectively as mucosa-associated lymphoid tissue (MALT). The gut-associated lymphoid tissue (GALT) includes the tonsils, adenoids, and the appendix and Peyer’s patches of the gastrointestinal tract. Similar, but less organized, aggregates of secondary lymphoid tissue line the respiratory epithelium, forming bronchial-associated lymphoid tissue (BALT). Additional, more diffuse lymphoid tissues are also found in most mucosae.
Although different from lymph nodes and spleen in outward appearance, the mucosal lymphoid tissues have similar microanatomy (Figure 1.28) and the function of trapping pathogens to activate lymphocytes. The differences are chiefly in the route of pathogen entry and the migration patterns of their lymphocytes (mucosal immunity is discussed in Chapter 10). Pathogens arrive at mucosa-associated lymphoid tissues by direct delivery across the mucosa, mediated by specialized M cells of the mucosal epithelium. Lymphocytes first enter mucosal lymphoid tissue from the blood. If they are not activated by specific antigen, the lymphocytes leave in lymphatics that connect the mucosal tissues to draining lymph nodes. Lymphocytes activated in mucosal tissues tend to stay within the mucosal system. Effector cells can move out of the lymphoid tissue and into the lamina propria and the mucosal epithelium to eliminate pathogens and counter infection. Alternatively, effector cells can recirculate in the blood and then return to mucosal tissue.
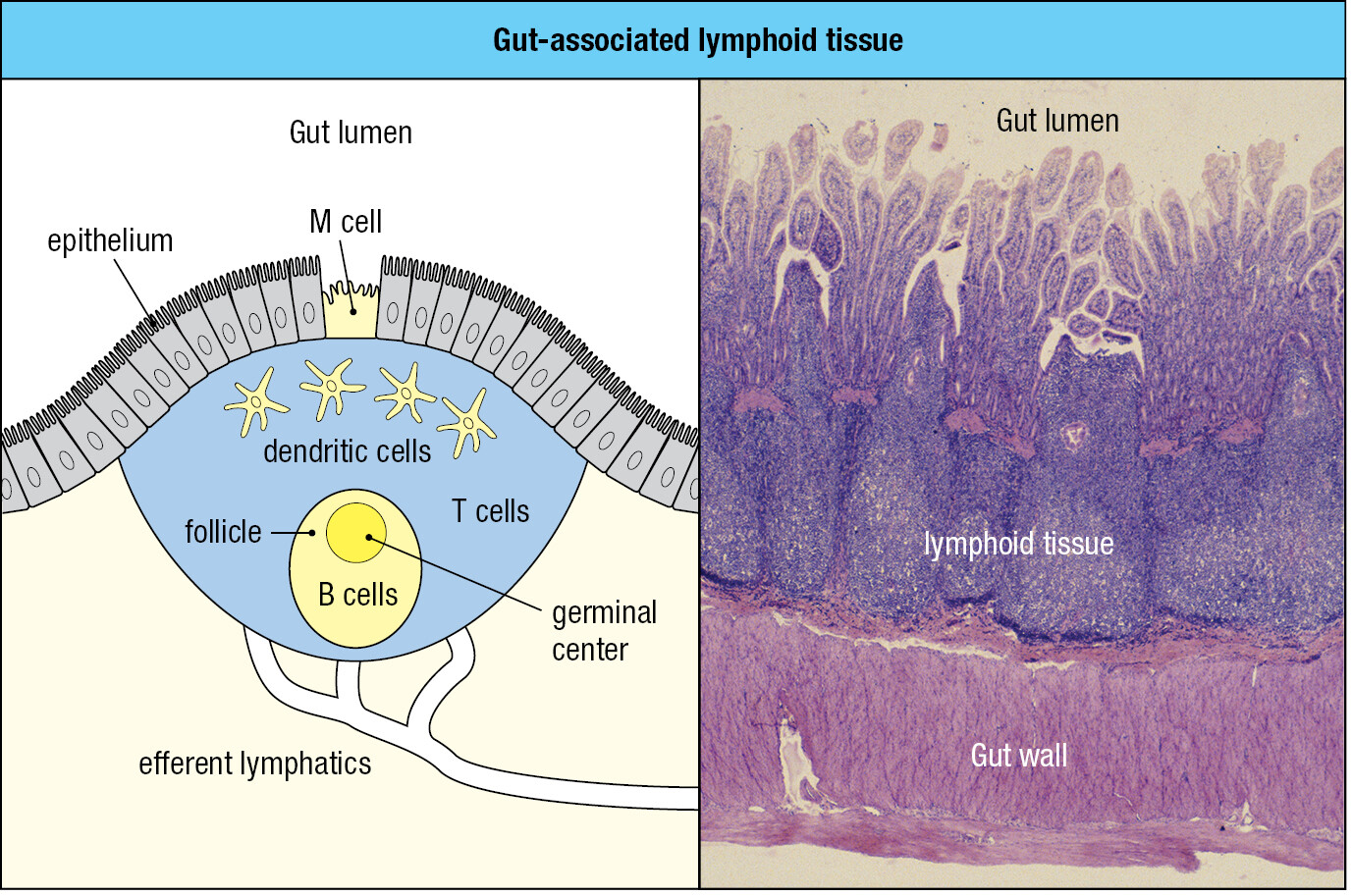
Glossary
- immune
- resistant to infection. (Chapter 1)
- immune system
- the tissues, cells, and molecules involved in the defense of the body against infectious agents. (Chapter 1)
- immunity
- the ability to resist a specific infection. (Chapter 1)
- vaccination
- the deliberate induction of protective immunity to a pathogen by the administration of killed or nonpathogenic forms of the pathogen or its antigens to induce an immune response. (Chapter 1, Chapter 11)
- commensal microorganisms
- a microorganism that habitually lives on or in the human body; one that normally causes no disease or harm and can be beneficial. (Chapter 1, Chapter 2)
- microbiota
- the microorganisms that habitually live in or on the human body; they normally do not cause disease and in many cases provide positive benefits for human health. (Chapter 1, Chapter 2)
- pathogen
- an organism, most commonly a microorganism, that can cause disease. (Chapter 1)
- opportunistic pathogens
- a microorganism that causes disease only in individuals whose immune systems are in some way compromised. (Chapter 1, Chapter 13)
- viruses
- submicroscopic pathogen composed of a nucleic acid genome enclosed in a protein coat. Viruses replicate only inside living cells because they do not possess all the metabolic machinery required for independent life. A viral particle is called a virion. (Chapter 1)
- bacteria
- (singular bacterium) diverse prokaryotic microorganisms that are responsible for many infectious diseases of humans and other animals. Some bacterial pathogens live only extracellularly, colonizing tissue surfaces and intercellular spaces; others can invade cells and live intracellularly. (Chapter 1)
- fungi
- a group of single-celled and multicellular eukaryot-ic organisms, including the yeasts and molds, that cause a variety of diseases. Immunity to fungi involves both antibody-mediated and cell-mediated responses. (Chapter 1)
- parasites
- general name for the unicellular protozoa and multicellular worms that infect animals and humans and live within them, causing disease. (Chapter 1)
- epithelium
- general name for a type of supracellular structure composed of a single layer (for example, the lining of the gut and respiratory tract) or multiple layers (for example, the epidermis) of cells bound tightly to each other. They are generally found as surface layers that communicate between the rest of a tissue and its environment. Epithelia line the internal and external cavities of the body and are also part of many internal organs. (Chapter 1)
- mucosal surfaces
- see mucosa. (Chapter 1)
- mucosae
- mucus-secreting epithelium such as that lining the respiratory, intestinal, and urogenital tracts. The mammary glands and the conjunctiva of the eye are also placed in this category. Mucosal epithelium communicates with the external environment and is the route of entry for most pathogens. (Chapter 1, Chapter 10)
- mucus
- slimy protective secretion composed of glycoproteins, proteoglycans, peptides, and enzymes that is produced by the goblet cells in many internal epithelia. (Chapter 1, Chapter 10)
- antimicrobial peptides
- any of a large number of small peptides produced by epithelial tissue that are toxic to microbes. Examples are the defensins. (Chapter 1)
- innate immune response
- immune response that is initiated immediately on infection and does not depend on lymphocytes of adaptive immunity. It depends on host defenses such as complement, neutrophils, macrophages, and NK cells, which provide nonspecific defense against a wide range of pathogens. This response does not generate immunological memory. See also induced innate immune response. (Chapter 1)
- effector mechanisms
- any of the physiological and cellular processes used by the immune system to destroy pathogens and remove them from the body. (Chapter 1)
- effector cells
- any of the terminally differentiated cells in an innate or adaptive immune response that are responsible for killing pathogens or removing them from the body. In the innate immune response these are mainly neutrophils, natural killer cells, and innate lymphocytes. In the adaptive immune response they are the antibody-producing plasma cells and the terminally differentiated classes of T cells—cytotoxic T cells, and TH1, TH2, TH17, TFH, and Treg cells. (Chapter 1)
- complement
- collection of plasma proteins that act in a cascade of reactions to attack extracellular forms of pathogens in extracellular spaces and the blood. Pathogens become coated with complement proteins, which can either kill the pathogen directly or facilitate its engulfment and destruction by phagocytes. It is involved in both innate and adaptive immunity and is activated either directly or indirectly by the presence of infection. (Chapter 1, Chapter 2)
- innate immunity
- the host defense mechanisms that act from the start of an infection and do not adapt to a particular pathogen or generate immunological memory. See also induced innate immune response. (Chapter 1, Chapter 2)
- cytokines
- any of a large number of small proteins secreted by cells that act locally to change the behavior of neighboring cells. Cytokines act by binding to specific receptors on their target cells. Cytokines made by lymphocytes and other immune-system cells are often called interleukins (abbreviated IL). (Chapter 1, Chapter 3)
- inflammation
- general term for the local accumulation of fluid, plasma proteins, and white blood cells that is caused by physical injury, infection, or a local immune response. It is also called an inflammatory response. The cells that invade tissues and help mediate inflammation are often called inflammatory cells. Those cytokines that promote inflammation are known as inflammatory cytokines. (Chapter 1, Chapter 3)
- endothelium
- epithelium lining the interior of blood vessels. (Chapter 1)
- edema
- abnormal accumulation of fluid in connective tissue, leading to swelling. (Chapter 1)
- inflammatory cells
- see inflammation. (Chapter 1)
- lymphocytes
- general name for a lineage of white blood cells consisting of several cell types. B lymphocytes (B cells) and T lymphocytes (T cells) are small cells that bear variable cell-surface receptors for antigen and are responsible for adaptive immune responses. Natural killer cells (NK cells) and the other innate lymphoid cells (ILCs 1, 2, and 3, and LTi cells) are large cells and do not bear variable antigen receptors. They are the lymphocytes of innate immunity. (Chapter 1)
- adaptive immune response
- the response of antigen-specific B and T lymphocytes to antigen, including the development of immunological memory. (Chapter 1)
- adaptive immunity
- the state of resistance to infection that is produced by the adaptive immune response. (Chapter 1)
- clonal selection
- the central principle of adaptive immunity. It is the mechanism by which adaptive immune responses derive only from individual antigen-specific lymphocytes, which are stimulated by the antigen to proliferate and differentiate into antigen-specific effector cells. (Chapter 1, Chapter 4)
- clonal expansion
- the multiplication of lymphocytes after their activation by antigen, so that large clones of rare antigen-specific lymphocytes are generated to fight the infecting pathogen. (Chapter 1)
- immunological memory
- the capacity of the immune system to make quicker and stronger adaptive immune responses to successive encounters with an antigen. It is specific for a particular antigen and is long-lived. (Chapter 1)
- memory cells
- general term for a lymphocyte that is responsible for the phenomenon of immunological memory. (Chapter 1, Chapter 11)
- acquired immunity
- alternative term for adaptive immunity; pathogen-specific immunity acquired as a consequence of infection or vaccination. (Chapter 1)
- protective immunity
- the specific immunological resistance to a pathogen that is present in an individual during months after either vaccination or recovery from an infection with the pathogen, and which is due to pathogen-specific antibodies and effector T cells produced during the primary response. (Chapter 1, Chapter 11)
- primary immune response
- the adaptive immune response that follows a person’s first exposure to an antigen. (Chapter 1,Chapter 6)
- secondary immune response
- the adaptive immune response provoked by a second exposure to an antigen. It differs from the primary response by starting sooner and building more quickly, and is due to the presence of long-lived memory B cells and T cells specific for the antigen. (Chapter 1, Chapter 11)
- leukocytes
- general term for a white blood cell. Lymphocytes, granulocytes, and monocytes are all leukocytes. (Chapter 1)
- hematopoiesis
- the generation of the cellular elements of blood, including the red blood cells, white blood cells, and platelets. These cells all originate from pluripotent hematopoietic stem cells whose differentiated progeny divide under the influence of various hematopoietic growth factors. (Chapter 1)
- pluripotent hematopoietic stem cell
- the stem cell in bone marrow that gives rise to all the cellular elements of the blood. (Chapter 1)
- erythrocytes
- red blood cell. (Chapter 1)
- megakaryocytes
- large cell of the erythroid lineage, produced in the bone marrow and resident there, from which blood platelets are produced by fragmentation. (Chapter 1)
- platelets
- a small non-nucleated cell fragment, derived from a megakaryocyte, that is present in large numbers in the blood and is essential for blood clotting. (Chapter 1)
- hematopoietic cells
- any blood cell or blood-cell precursor. (Chapter 1)
- bone marrow
- tissue in the center of certain bones that is the major site of generation of all the cellular elements of the blood (hematopoiesis). (Chapter 1)
- self renewal
- the ability of a population of cells to maintain itself permanently by mitosis. In the case of stem cells, self renewal involves mitosis and limited differentiation. (Chapter 1)
- myeloid precursor cell
- the stem cell in bone marrow that gives rise to the myeloid lineage (granulocytes, monocytes, macrophages, mast cells, and dendritic cells). (Chapter 1)
- myeloid cell lineage
- the granulocytes, monocytes, macrophages, mast cells and dendritic cells, and the bone marrow cells that give rise to them. (Chapter 1)
- granulocytes
- white blood cell with irregularly shaped, multilobed nuclei, and cytoplasmic granules. There are three types of granulocyte: neutrophil, eosinophil, and basophil. Granulocytes are also called polymorphonuclear leukocytes. (Chapter 1)
- neutrophils
- phagocytic white blood cell that enters infected tissues in large numbers and engulfs and kills extracellular pathogens. It is a type of granulocyte and contains granules that stain with neutral dyes; hence its name. It is by far the most abundant white blood cell. (Chapter 1, Chapter 3)
- eosinophils
- white blood cell that is one of the three types of granulocyte. It contains granules that stain with eosin (hence its name) and whose contents are secreted when the cell is stimulated. Eosinophils contribute chiefly to defense against parasitic infections. (Chapter 1, Chapter 14)
- basophils
- white blood cell present in small numbers in the blood; it is one of the three types of granulocyte. Basophils contain granules that stain with basic dyes; hence their name. (Chapter 1, Chapter 14)
- polymorphonuclear leukocytes
- an alternative name for the granulocyte (a class of white blood cells comprising neutrophils, eosinophils, and basophils), describing the varied morphology of the nuclei. (Chapter 1, Chapter 3)
- phagocytosis
- the engulfment and degradation of extracellular material, such as microorganisms, dead cells, and macromolecules, by specialized cells called phagocytes. The principal phagocytes involved in immune responses are macrophages, neutrophils, and dendritic cells. See also receptor-mediated endocytosis. (Chapter 1, Chapter 3)
- phagocytes
- a cell specialized to perform phagocytosis. The principal phagocytic cells in mammals are neutrophils and macrophages. (Chapter 1, Chapter 3)
- pus
- thick yellowish-white fluid that is formed in infected wounds. It is composed of dead and dying white blood cells (principally neutrophils), tissue debris, and dead microorganisms. (Chapter 1, Chapter 3)
- macrophage
- large mononuclear phagocytic cell resident in most tissues that bears receptors for many components of pathogens. Tissue macrophages are initially embryonic in origin (embryonic macrophages) but can be supplemented throughout life by macrophages derived from blood monocytes (hematopoietic macrophages). They contribute to innate immunity and early nonadaptive phases of host defense, as well as being effector cells in adaptive immune responses. They function as general phagocytic scavenger cells and as frontline sensors of infection, producing cytokines that recruit a variety of immune-system cells to an ongoing immune response. They can also function as professional antigen-presenting cells. (Chapter 1, Chapter 3)
- tissue-resident macrophages
- self-renewing macrophage that derives from an embryonic stem cell and comes to reside within tissues during embryonic development. These macrophages of embryonic origin can be supplemented during life by hematopoietic macrophages derived from blood monocytes. (Chapter 1)
- monocytes
- phagocytic white blood cell with a bean-shaped nucleus. It is the precursor of the hematopoietic macrophage. (Chapter 1)
- dendritic cells
- professional antigen-presenting cell with a branched, dendrite-like morphology that is present in tissues. It is derived from the bone marrow and is distinct from the follicular dendritic cell that presents antigen to B cells. Immature dendritic cells take up and process antigens but cannot yet stimulate T cells. Mature or activated dendritic cells are present in secondary lymphoid tissues and are able to stimulate T cells. Dendritic cells form a bridge between the innate and adaptive immune responses, as interactions between dendritic cells and NK cells are important in determining whether an adaptive immune response needs to be made. (Chapter 1, Chapter 3)
- mast cell
- large cell derived from bone marrow and resident in connective tissues throughout the body. Mast cells contain large granules that store a variety of chemical mediators including histamine. Mast cells have high-affinity Fcε receptors (FcεRI) that bind free IgE. Antigen binding to IgE associated with mast cells triggers mast-cell activation and degranulation, producing a local or systemic immediate hypersensitivity reaction. Mast cells have a crucial role in allergic reactions and anti-parasite immunity. (Chapter 1)
- lymphoid precursor cell
- stem cell in bone marrow that gives rise to all types of lymphocyte (the lymphoid lineage). (Chapter 1)
- lymphoid lineage
- all types of lymphocyte, and the bone marrow cells that give rise to them. (Chapter 1)
- large granular lymphocytes
- an alternative and earlier name for the natural killer cell (NK cell). Microscopy revealed two types of blood lymphocyte: the small lymphocytes (B cells and T cells) and the large granular lymphocytes (NK cells). (Chapter 1)
- natural killer cells (NK cells)
- large, granular, cytotoxic lymphocyte that circulates in the blood and is central to the innate immune response to intracellular pathogens, notably viruses. NK cells do not have the equivalent of Ig or T-cell receptors but have numerous other receptors by which they recognize and kill virus-infected cells and tumor cells. (Chapter 1, Chapter 3)
- small lymphocytes
- the general name for a recirculating resting T or B lymphocyte. (Chapter 1)
- B lymphocytes
- alternative name for B cell. (Chapter 1)
- T lymphocyte
- alternative name for T cell. (Chapter 1, Chapter 5, Chapter 7)
- B cells
- one of the two main types of lymphocyte responsible for adaptive immunity (the other is the T cell). The B-cell arm of the immune system is dedicated to making immunoglobulins in the form of cell-surface antigen receptors (the B-cell receptors) and secreted antibodies. Also called a B lymphocyte. (Chapter 1)
- T cells
- one of the two main classes of lymphocyte responsible for adaptive immunity (the other is the B cell). T cells originate in the bone marrow, develop in the thymus, and are responsible for cell-mediated immunity. Their cell-surface antigen receptor is called the T-cell receptor. Effector T cells comprise various subtypes including the cytotoxic T cell (which is responsible for killing virus-infected cells), the regulatory T cell, and a number of subsets of helper T cells that cooperate with other immune-system cells to aid macrophage activation and antibody production. Also called T lymphocyte. (Chapter 1, Chapter 5, Chapter 7)
- B-cell receptors
- one of the two main types of lymphocyte responsible for adaptive immunity (the other is the T cell). The B-cell arm of the immune system is dedicated to making immunoglobulins in the form of cell-surface antigen receptors (the B-cell receptors) and secreted antibodies. Also called a B lymphocyte. (Chapter 1)
- immunoglobulins
- the general name for antibodies and B-cell antigen receptors. (Chapter 1, Chapter 4)
- T-cell receptors
- the highly variable antigen receptor of T lymphocytes. On most circulating T cells it is composed of a variable α chain and a variable β chain and is known as the αβ T-cell receptor. This receptor recognizes peptide antigens derived from the breakdown of proteins. A minority of circulating T cells carry a γδ T-cell receptor, in which the two chains are called γ and δ and are generally less diverse in their specificity and can recognize antigens other than peptides, such as phosphoantigens and glycolipids. Both types of receptor are present at the cell surface in association with a complex of invariant CD3 chains and ζ chains, which have a signaling function. (Chapter 1, Chapter 5)
- plasma cells
- terminally differentiated B lymphocyte that is dedicated to the synthesis and secretion of antibodies. (Chapter 1, Chapter 4)
- antibody
- the secreted form of the immunoglobulin expressed by a B cell. As a result of V(D)J recombination, each mature B cell expresses immunoglobulin with a different specificity for antigen. Antibodies are produced by the plasma cells that differentiate from B cells after activation by antigen. (Chapter 1, Chapter 4)
- antigen
- any molecule or molecular fragment that either is recognized by an antibody or B-cell receptor or can be bound by an MHC molecule and presented to a T-cell receptor. See also epitope. (Chapter 1)
- antigen receptors
- the highly variable cell-surface receptor on lymphocytes that recognizes antigen. For a B cell, the receptor is a cell-surface immunoglobulin; for a T cell, the receptor is a rather similar molecule called the T-cell receptor. All the antigen receptors on an individual B or T lymphocyte are identical and recognize the same antigen epitope. (Chapter 1)
- cytotoxic T cells
- type of effector T cell that kills its target cells. It expresses the CD8 co-receptor and recognizes peptide antigens presented by MHC class I molecules. It is important in host defense against viruses and other cytosolic pathogens because it can recognize and kill the infected cells. (Chapter 1, Chapter 5)
- helper T cells
- general name for effector CD4 T cells that function to help other immune-system cells perform their roles, such as to help B cells produce antibody. (Chapter 1, Chapter 5)
- TH1 cell
- a type of effector CD4 T cell that is characterized by the production of inflammatory cytokines. TH1 cells are involved mainly in activating macrophages. Also known as inflammatory T cell. (Chapter 1, Chapter 8)
- TH17 cell
- a type of effector CD4 T cell that is characterized by the production of IL-17 and the promotion of inflammatory responses. The activities of these cells are associated with autoimmune conditions. (Chapter 1, Chapter 8)
- TH2 cell
- a type of effector CD4 T cell that is characterized by the production of noninflammatory cytokines that promote the production of IgE antibodies. (Chapter 1, Chapter 8)
- TFH cell
- see T follicular helper cell. (Chapter 8)
- Treg cell
- see regulatory T cell. (Chapter 1, Chapter 7, Chapter 8)
- epitope
- the portion of an antigenic molecule that is bound by an antibody or gives rise to the MHC-binding peptide that is recognized by a T-cell receptor. Also called an antigenic determinant. (Chapter 1, Chapter 4)
- major histocompatibility complex molecule (MHC molecule)
- any of the MHC class I or class II proteins, which are encoded in the major histocompatibility complex. (Chapter 1, Chapter 5)
- MHC molecule
- any of the MHC class I or class II proteins, which are encoded in the major histocompatibility complex. (Chapter 1, Chapter 5)
- neutralization
- the mechanism by which antibodies binding to sites on pathogens prevent growth of the pathogen and/or its entry into cells. The toxicity of bacterial toxins can similarly be neutralized by bound antibody. (Chapter 1)
- humoral immunity
- immunity that is mediated by antibodies and can therefore be transferred to a nonimmune recipient by serum. (Chapter 1, Chapter 8)
- humors
- old-fashioned term for the body fluids. (Chapter 1)
- opsonization
- the coating of the surface of a pathogen or other particle with any molecule that makes it more readily ingested by phagocytes. Antibody and complement opsonize extracellular bacteria for phagocytosis by neutrophils and macrophages because the phagocytic cells carry receptors for these molecules. (Chapter 1, Chapter 2)
- lymphoid tissues
- see tertiary lymphoid structure. (Chapter 16)
- primary lymphoid tissues
- anatomical site of lymphocyte development. In humans, these are the bone marrow (where B lymphocytes develop) and the thymus (where T lymphocytes develop from precursor cells that have migrated from the bone marrow). Also known as central lymphoid tissue. (Chapter 1)
- thymus
- a primary lymphoid organ situated in the upper part of the middle of the chest, just behind the breastbone. It is the site of T-cell development. (Chapter 1, Chapter 7)
- secondary lymphoid tissues
- the lymph nodes, spleen, and mucosa-associated lymphoid tissues. These are the tissues in which immune responses are initiated. The highly organized tissues such as lymph nodes and spleen are also known as secondary lymphoid organs. Also known as peripheral lymphoid tissue. (Chapter 1)
- lymphatic vessels
- thin-walled vessel that carries lymph. Often simply called a lymphatic. Lymphatic vessels are part of the lymphatic system that transports lymph from tissues to secondary lymphoid tissues (with the exception of the spleen) and from secondary lymphoid tissues to the thoracic duct. (Chapter 1)
- lymphatics
- see lymphatic vessel. (Chapter 1)
- lymph
- mixture of extracellular fluid and cells that is carried by the lymphatic system. (Chapter 1)
- lymphocyte recirculation
- the continual migration of naive lymphocytes from blood to secondary lymphoid tissues to lymph and back to the blood. An exception to this pattern of traffic occurs in the spleen: lymphocytes both enter and leave the spleen in the blood. (Chapter 1)
- lymph node
- a bean-shaped structure, a secondary lymphoid tissue present at many sites in the body where lymphatic vessels converge. Antigens are delivered by the lymph and presented to lymphocytes within the lymph node to initiate adaptive immune responses. (Chapter 1)
- draining lymph node
- the lymph node nearest to a site of infection, to which extracellular fluid containing antigen and cells from the site is transported. (Chapter 1)
- lymphoid follicles
- an aggregation of mainly B cells in secondary lymphoid tissues. Naive B cells must pass through follicles for their survival; after B cells have been activated by antigen they enter the follicles, where they proliferate and undergo somatic hypermutation and isotype switching. (Chapter 1)
- afferent lymphatic vessel
- vessel that brings lymph into a lymph node. (Chapter 1)
- efferent lymphatic vessel
- vessel by which lymph and lymphocytes leave a lymph node en route to the blood. (Chapter 1)
- germinal center
- area in secondary lymphoid tissue that is a site of intense B-cell proliferation, selection, maturation, and cell death. Germinal centers form around follicular dendritic cell networks when activated B cells migrate into lymphoid follicles. The cellular and morphological events that form the germinal center and take place there are called the germinal center reaction. (Chapter 1, Chapter 6, Chapter 9)
- spleen
- an organ situated adjacent to the cardiac end of the stomach. One function of the spleen is to remove old or damaged red blood cells from the circulation; its other function is as a secondary lymphoid organ in which immune responses to blood-borne pathogens and antigens are initiated. (Chapter 1)
- immunodeficiency disease
- any inherited or acquired disorder in which some part or parts of the immune system are either absent or defective, resulting in failure to mount an effective immune response to pathogens. (Chapter 1, Chapter 13)
- mucosa-associated lymphoid tissue (MALT)
- aggregations of lymphoid cells in mucosal epithelia and in the lamina propria beneath. The main mucosa-associated lymphoid tissues are the gut-associated lymphoid tissue (GALT) and the bronchial-associated lymphoid tissue (BALT). (Chapter 1)
- gut-associated lymphoid tissue (GALT)
- all lymphoid tissue closely associated with the gastrointestinal tract, including the palatine tonsils, Peyer’s patches in the intestine, isolated B-cell follicles, and intraepithelial lymphocytes. (Chapter 1, Chapter 10)
- tonsils
- large aggregates of lymphoid cells lying on each side of the pharynx. (Chapter 1)
- adenoids
- mucosa-associated secondary lymphoid tissues located in the nasal cavity. (Chapter 1, Chapter 10)
- appendix
- gut-associated secondary lymphoid tissue located at the beginning of the colon. (Chapter 1)
- Peyer’s patches
- organized gut-associated lymphoid tissue present in the wall of the small intestine, especially the ileum. (Chapter 1, Chapter 10)
- bronchial-associated lymphoid tissue (BALT)
- the lymphoid cells and organized lymphoid tissues of the respiratory tract. (Chapter 1)
- M cells
- microfold cell, a specialized cell type in intestinal epithelium through which antigens and pathogens enter gut-associated lymphoid tissue from the intestines. (Chapter 1, Chapter 10)